Research - (2022) Volume 12, Issue 6
Carbon and nitrogen isotope composition and epicuticular wax of durum wheat (Triticum durum Desf.) plants exposed to water deficit at anthesis
S. Chahbar1*, D. Mehdeb2, M. Ouis3 and J.L. Araus4Abstract
Various morphological and physiological characters related to the water deficit were studied at five durum wheat (Triticum durum Desf.) genotypes under three water regimes (well-water, 60% PC and 30% PC). Stomatal resistance (Rs), cuticular transpiration (RWL), relative water content (RWC) were measured in the flag leaf just before harvest. Chlorophyll content, soluble sugar and the chemical composition of epicuticular waxes of flag leaf and spike were determined. Specific leaf dry weight (SLDW), shoot dry weight (SDW), root shoot ratio (RD/SD), δ15N, δ13C were evaluated. The results indicated that water deficit involves many changes in durum wheat genotypes resulting in decreased in SLDW, δ15N, RWL, RWC and chlorophyll content and increase in δ13C, total wax, soluble sugar, stomatal resistance (Rs). Negatives correlations were showed between carbon isotope composition and SLDW as well as with chlorophyll content. A positive correlation was found between carbon isotope composition and stomatal resistance. The results also showed that epicuticular waxes had similar compositions in both organs except for certain constituents. Acetates, hydrocarbons, fatty acids, alkanes, bicyclical alkanes, alkenes, alcohols, aldehydes, ketones, cholesterols, esters, ethers, heterocyclicals and triterpenes were the main determined compounds.
Keywords
Durum wheat, Water stress, epicuticular wax, δ15N, δ13C, leaf, spike.
Introduction
Durum wheat (Triticum durum Desf.) is an important food for more than 35% of world population and it is the major source of calories (Kazemi, 2009). Generally has inextensible gluten and therefore, most of the durum wheat produced worldwide is milled into semolina to make a compact and stiff dough to manufacture alimentary pasta (Ammar et al., 2000). In West Asia and North Africa, durum wheat is used extensively to prepare regional foods such as couscous, frekeh, and bulgur. A recent increase in the world durum wheat production is not sufficient to meet the demands of a growing population and durum wheat production in many regions of the world is below average because of adverse environmental conditions and wheat cultivation is mainly restricted to such zones with scarcity of water (Gonzalez et al., 2010). Roughly 230 million ha of land is used for wheat cultivation worldwide and 50% of this area is usually afflicted with drought stress (Monneveux et al., 2006; Trethowan and Reynolds, 2006).
Durum wheat is widely cultivated in the Mediterranean regions where drought and high temperatures are two major environmental constraints limiting yield (Maccaferri et al., 2008) and where the climate is characterized by low and variable rainfall (Nachit et al., 2001).
Drought is generally accepted to be the most widespread abiotic stress experienced by crops, and is becoming an increasingly serious problem in many regions of the world (Boutraa et al., 2010).
The impact of drought combined with heat severely limit yield in the Mediterranean regions (Loss et al., 1994). Therefore, drought stress is a serious problem for durum wheat production in these areas, because it affects simultaneously many traits through morphological, physiological and metabolic changes occurring in all plant organs (Merah et al., 2001; Araus et al., 2003). Under water deficit conditions durum wheat decrease shoot growth in order to limit transpiration (Misra, 2010; Yasir et al., 2013).
Drought stress induces physiological and biochemical responses in plants. A wide range of strategies, which have been used to enhance the tolerance to drought depend on the genetically determined plant capacity and sensitivity, as well as on the intensity and duration of the stress (Jacobsen et al., 2012). Understanding the physiological and biochemical mechanisms providing drought tolerance is very important in terms of developing selection and breeding strategies (Shangguan et al., 2000; Monneveux et al., 2006; Araus et al., 2014).
Chlorophyll concentration has been known as an index for evaluation of source (Herzog, 1986), therefore decrease of this can be consideration as a nonstomata limiting factor in the drought stress conditions (Keyvan, 2010). There are reports about decrease of chlorophyll in the drought stress conditions (Ganji et al., 2012). Also, it is reported that chlorophyll content of resistant and sensitive cultivars to drought and thermal stress reduced. But resistant cultivar to drought and thermal stress conditions had high chlorophyll content (Zaefyzadeh et al., 2009; Chahbar et al., 2016). Vendruscoloa et al. (2011) reported that drought stress will reduce concentration of chlorophyll b more than chlorophyll a.
The carbon isotope composition of plant dry matter (δ13C), which is frequently expressed as the discrimination value (Δ13C), provides a time-integrated measurement of the plant’s transpiration efficiency over the period during which dry matter is assimilated; thus, this parameter has been proposed to be an indicator of water use efficiency (Farquhar and Richards, 1984; Farquhar et al., 1989). In C3 plants, Δ13C has been used to evaluate drought stress (Araus et al., 2013) because, under conditions in which the plant is not water-stressed, the stomatal conductance is high and CO2 diffusion in and out of the leaf is relatively free.
Plants regulate stomatal conductance to optimize carbon uptake with respect to water loss (Farquhar et al., 1980a). An important limitation in this process is the rate at which stomata open in the light or close under darkness or water deficit (Lawson et al., 2011; Vico et al., 2011). However, although stomatal response are known to vary widely across species (Vico et al., 2011), the biophysical factors governing the rate of response are not well understood.
The plant cuticle is a continuous lipophilic layer covering the surface of all epidermal cell types and is one of their distinctive characteristics (Javelle et al., 2011). It forms a vital hydrophobic barrier over the aerial surfaces of land plants during primary stages of development, limiting nonstomatal water loss and gaseous exchanges, controlling the absorption of lipophilic compounds, providing mechanical strength and visco elastic properties (Lu et al., 2009; Jenks et al., 2010), preventing organ fusion during development (Sieber et al., 2000), as well as protecting the plant from nonbiotic and biotic stressors from the environment (schoppach et al., 2012). During water stress, when stomata are closed, plant survival depends on the amount of water lost through the cuticle. From a whole-plant point of view, the interplay between stomatal regulation and cuticular water permeability is therefore essential (Karbulkova et al., 2008).
The cuticular transpiration, also termed residual transpiration, included the transpiration through the cuticle together with that due to an inadequate closing of guard cells (Araus et al., 1991; Merah, 2001; Ganzalez et al., 2010). Epicuticular waxes help leaves retain water by minimising cuticular transpiration (Vadez et al., 2014). As water becomes less available the stomata close to minimise transpiration losses; under such conditions the loss of water occurs mainly form the area between the stomata (Chahbar et al., 2016).
The objective of this study is to investigate the effect of water stress on growth of five durum wheat cultivars. A number of growth parameters were determined under three water deficit regimes (30, 60 and 100% PC), including relative water content (RWC), cuticular transpiration, quantitative and qualitative wax composition, stomatal resistance, pigment content, soluble sugar, carbon and nitrogen isotope composition, specific leaf dry weight, shoot dry weight, and ratio RD/SD.
Materials and Methods
Experiments and growing conditions
Experiment consisted of 5 durum wheat (Triticum durum Desf.) genotypes, obtained from the Technical Institute of Field Crops (ITGC, Tiaret. Algeria), and selected on the basis of growth analysis and differential responses to water stress, They include Two Algerian genotypes (Bidi17 and Hedba3) known as locally adapted and are characterized by low productivity and low yield stability. In addition one cultivar (Acsad 1277) from the Arabian Centre for Studies of Arid Zones and Drylands (ACSAD), one cultivar (Waha) from the International Center for Agricultural Research in the Dry Areas (ICARDA) and one cultivar (Chen’s) from International Maize and Wheat Improvement Center (CIMMYT) were included.
The experiment was grown in a greenhouse at the Laboratory of Plant Physiology of University Ibn Khaldoun of Tiaret (West of Algeria, 35.33 35° 19'N, 1.333 1° 19'E and 1075m elevation). Equipped with a ventilation system that maintained the temperature at about 25/18°C (day/night) and the relative humidity at 70/80% (day/night).
Seeds were surface sterilized with sodium chloride, washed then germinated on soaked filter paper in petri dishes. After the emergence of the first leaf, only one plant per pot was left in PVC cylinders (60 cm height and 10 cm diameter) filled with a mixture of sand, soil and organic dry matter (8:1:1).
After sowing, control plants were irrigated to keep pots to 100% pot capacity (WW) for a period of 4 months. In the stress treatments pots were irrigated up to 100% of the pot capacity (PC) until 4-5 leaf stage and then watering was decreased to reach 60% of pot capacity for mild water stress (60%PC) and 30% pot capacity (30%PC) for severe water stress until anthesis then maintained one week. Water was added every 2-3 days if needed. The process involved weighing each pot and then applying the required amount of water. The experimental design involved a randomized complete block with three water regimes, five genotypes and three replicates per genotype and growing condition. In the present study plants were grown under different treatments assayed until anthesis, when different physiological determinations and sampling were measured before harvesting.
Measurements
Leaf physiological measurements were conducted one week after anthesis (one week before harvest). Stomatal resistance (Rs), cuticular transpiration (RWL), relative water content (RWC) and specific leaf dry weight (SLDW) were measured in the same flag leaf at 12:00 a.m. Waxes, pigment content and soluble sugar were extracted in flag leaf before harvest.
Stomatal resistance
Stomatal resistance (Rs, s.cm-1) was measured on flag leaves, using porometer (Ap4 Delata-T devices cambridge UK. The measurements were taken around.
Cuticular transpiration
Cuticular transpiration was measured according to Clarke et al. (1991) on excised flag leaves. Flag leaves were taken at anthesis, After cutting, the flag leaf was immediately immersed in distilled water inside of a glass tube for 6h at 4°C, which was immediately weighed to determined turgid weight (TW) using a precision balance. The leaves were then placed in a controlled environment chamber in the dark at 25°C and at a relative humidity of 50%. Weighted again after 120 min to estimate water loss by cuticular transpiration. The dry weights (DW) were obtained after oven drying the leaves at 80°C during 48 h. Water loss was expressed in gram of water lost per gram of leaf dry matter.
Relative water content
Plant water status was determined at anthesis by measuring the relative water content (RWC) of flag leaves. The relative water content was determined using the method of Sangakkara et al. (1996) as: 100 × (FW-DW)/(TW-DW) where Fw is fresh weight, DW is dry weight and TW is turgid weight after re-hydrating the leaves. The leaves were kept in distilled water in a closed glass flask at 5°C in darkness (to minimize respiration losses) until they reached a constant weight. The FW of the leaves was determined immediately after collecting samples. Leaves were then dried for 48 h at 80°C to determine DW. Three replicates per cultivar and treatment were obtained.
Soluble sugars
Soluble sugars were extracted in 80% ethanol from 100 mg of flag leaf fresh tissue and quantified by the classical anthrone method of Shieds and Burnet (1960) using a spectrophotometer (Pharmacia Biotech. Novaspec II, Ontario, Canada). A standard curve was established using glucose and the results are therefore expressed in μg.g-1 of fresh weight (Fw).
Chlorophyll content
For chlorophyll content, 1 g of tissue was extracted with 10 ml of acetone (95%); chlorophyll a, b and carotenoids were specifically quantified according to Lichtenthaler (1987) and Shabala et al. (1998).
Chl a=9.784 × Do (662)-0.99 × Do (644)
Chl b=21.42 × Do (644)-4.65 × Do (662)
Total chlorophyll=Chl a+Chl b
Carot(x+c)=(1000 DO (470)-1.90 Chl a-Chl b)/214
Od: Optical density
Wax analysis
The cuticular waxes were extracted by immersing whole flag leaf and spike two times for 30 s into 5 ml of chloroform (CHCl3) at room temperature. Both CHCl3 solutions were combined and n-tetracosane was added as internal standard. The solvent was removed under a gentle stream of nitrogen gas, and the remaining wax mixture was redissolved in 1 ml of CHCl3 and stored at 4°C until used. Prior to Gas Chromatography (GC) analysis, chloroform was evaporated from the samples under a gentle stream of nitrogen gas while heating to 50°C. Then the wax mixtures were treated with bis-N,N-(trimethylsilyl) trifluoroacetamide (BSTFA; Sigma) in pyridine (30 min at 70°C) to transform all hydroxyl-containing compounds into the corresponding trimethylsilyl derivatives.
Measurements of qualitative composition were conducted at the Scientific Facilities of the University of Barcelona, using GC. The quantitative composition of the mixtures was studied using capillary GC with flam ionization detector (FID) under the same GC conditions as above. Single compounds were quantified against the internal standard by automatically integrating peak areas. The total amount of cuticular waxes was expressed as per leaf area according to Jenks et al. (2000).
Shoot biomass
After the different physiological measurements, the rest of the shoot was then harvested; oven dried at 80ºC for 48 h weighed to determined shoot dry weight (SDW) and finely ground for stable isotope analyses. The plants were uprooted, roots carefully separated from the soil and washed and dried at 80°C for 48 h. Subsequently the material was weighed in order to determine root dry weight. Finally, root to shoot ratio (RD/SD) was calculated.
Specific flag leaf dry weight (SLDW)
The specific flag leaf dry weight (SLDW) was calculated using the following formula.
SLDW (g.cm-2)=FLDW/FLA
Where FLDW is the flag leaf dry weight and FLA is the flag leaf area.
Stable carbon and nitrogen isotope
Stable carbon 13C/12C and nitrogen 15N/14N isotope ratios were determined in shoot dry of all the plants. Measurements of carbon and nitrogen were conducted at the Scientific Facilities of the University of Barcelona, using an element analyser (Flash 1112 EA; ThermoFinnigan, Berman Germany) coupled with an isotope ratio mass spectrometer. For each genotype, samples were dried at 80°C for 48 h, and ground to a fine powder. 1 mg and reference materials were weighed into tin capsules, sealed and loaded into an automatic sampler before EA-IRMS analysis. Isotopic results are expressed in standard δ-notation according to Coplen, 2008 as:
X=[(Rsample/Rstandard)-1] × 1000, where X is the δ15N or δ13C value, and R=15N/14N or 13C/12C, respectively. The δ15N values are reported relative to the standard N2 in air, whereas δ13C values are reported relative to the Vienna PeeDee Belemnite standard (Farquhar et al., 1989).
Statistical analyses
Data were subjected to analysis of variance (ANOVA). Effects of genotype, water regimes and their interaction were determined. Tukey’s b test was used to establish differences between genotypes. The analyses were made using SPSS. Pearson phenotypic correlations were calculated to determine the relationship between traits.
Results
The effect of water regime and genotype on the different studied traits
The effect of water regime and genotype on RWC, RWL and stomatal resistance (Rs): There were significant genotypic differences between durum wheat genotypes for all studied traits in Table 1 except for stomatal resistance. The increase in water stress significantly affected all studied traits. However, Water stress intensity strongly reduce stomatal resistance, this reduction was about 184.4% and 95.6% respectively under 30% and 60% PC compared with WW plants. Nevertheless, the impact of water regime was marked in Hedba 3 by 2.84 s.cm-1 (Table 1).
T | G | RWC (%) | RWL (g.cm-2 mn-1) | Rs (s.cm-1) |
---|---|---|---|---|
100% | Chen's | 90.11ab | 0.0055b | 1.84a |
Acsad | 89.06ab | 0.0054b | 2.54ab | |
Waha | 86.83a | 0.0028a | 2.64ab | |
Bidi 17 | 90.07ab | 0.0045ab | 3.67b | |
Hedba | 91.57b | 0.0030a | 2.25a | |
Means | 89.53 | 0.0042 | 2.59 | |
60% | Chen's | 84.96a | 0.0035a | 3.51a |
Acsad | 86.58a | 0.0046a | 7.13a | |
Waha | 82.03a | 0.0046a | 6.53a | |
Bidi 17 | 88.13a | 0.0019a | 5.28a | |
Hedba | 89.13a | 0.0013a | 2.85a | |
Means | 86.17 | 0.0032 | 5.06 | |
30% | Chen's | 76.34ab | 0.0032cd | 7.57a |
Acsad | 75.94ab | 0.0031abc | 8.07a | |
Waha | 67.50a | 0.0031c | 7.89a | |
Bidi 17 | 86.87b | 0.0017a | 7.47a | |
Hedba | 87.54b | 0.0021ab | 5.80a | |
Means | 78.84 | 0.0026 | 7.36 | |
ANOVA | G | 0.000 | 0.003 | ns |
T | 0.000 | 0.004 | 0.002 | |
G × T | ns | ns | ns |
RWC declined in the leaves under water stress by 78.84% under (30% PC) and 89.53% under (60% PC) compared with WW conditions. The decrease in leaf RWC was lowest in Waha by 67.50% and 82.03% at 60 and 30% PC respectively and highest in Hedba 3 with 87.53% and 89.13% at 60 and 30% PC respectively.
The decrease of water loss by RWL for the tested genotypes under control and the two water stress conditions is obvious. However, cultivars reacted differently to water stress. For example, cultivar Waha, which had the lowest RWL under no stress (0.0027 g.cm-2.mn-1), had one of the highest RWL under water stress (0.0046 and 0.0031 g.cm-2.mn-1) (Table 1).
Nevertheless, interactions between genotypes and water regimes (G x T) were not significant for all studied traits.
Effect of water regimes and genotypes on soluble sugar and pigment content: Significant genotypic differences existed for all parameters except soluble sugar and chla chlb ratio. Water regime significantly affected all the traits included in Table 2 except chla chl b ratio.
T | G | Soluble Sugar (μg.g-1F.w) |
chl a (μg.g-1F.w) |
chl b (μg.g-1F.w) |
Carot (μg.g-1F.w) |
tot chl (μg.g-1F.w) |
Chl a/chl b | tot chl/carot |
---|---|---|---|---|---|---|---|---|
100% | Chen's | 3.86a | 20.69c | 9.17b | 7.72c | 29.86c | 2.45a | 3.55ab |
Acsad | 3.18a | 22.74c | 11.92c | 7.37bc | 34.66c | 1.91a | 4.70c | |
Waha | 4.16a | 17.01bc | 9.75bc | 6.10ab | 26.76bc | 1.74a | 4.38bc | |
Bidi 17 | 3.95a | 10.70a | 3.41a | 5.22a | 14.10a | 3.18b | 2.70a | |
Hedba | 3.21a | 13.93ab | 5.96a | 4.86a | 19.89ab | 2.37a | 4.19bc | |
Means | 3.67 | 17.01 | 8.04 | 6.25 | 25.06 | 2.30 | 3.97 | |
60% | Chen's | 3.91a | 13.52b | 6.48b | 6.59c | 20.00ab | 2.05a | 2.95ab |
Acsad | 3.86a | 15.77b | 6.97b | 6.26c | 22.74b | 2.25a | 3.62b | |
Waha | 5.20a | 11.77ab | 3.73ab | 5.78bc | 15.50ab | 2.92a | 2.68ab | |
Bidi 17 | 5.00a | 8.36ab | 3.57ab | 3.57a | 11.92ab | 2.35a | 3.29ab | |
Hedba | 4.20a | 6.68a | 2.71a | 4.27ab | 9.39a | 2.42a | 2.07a | |
Means | 4.43 | 11.22 | 4.69 | 5.29 | 15.91 | 2.61 | 2.93 | |
30% | Chen's | 5.65a | 11.45a | 5.04a | 4.37ab | 16.50a | 2.21a | 3.69a |
Acsad | 6.63a | 12.72a | 4.79a | 4.85ab | 17.51a | 2.72a | 3.56a | |
Waha | 10.75a | 6.78a | 2.04a | 5.12b | 8.82a | 3.53a | 1.72a | |
Bidi 17 | 7.67a | 5.28a | 2.00a | 2.35a | 7.28a | 2.68a | 3.46a | |
Hedba | 7.02a | 4.33a | 1.80a | 2.55a | 6.12a | 2.29a | 2.62a | |
Means | 7.54 | 8.11 | 3.14 | 3.85 | 11.25 | 2.70 | 2.86 | |
ANOVA | G | ns | 0.000 | 0.000 | 0.000 | 0.000 | ns | 0.022 |
T | 0.000 | 0.000 | 0.000 | 0.000 | 0.000 | ns | 0.000 | |
G X T | ns | Ns | ns | ns | ns | ns | 0.034 |
Table 2. Effect of different levels of water regime and genotype on soluble sugar, pigment content (chlorophyll a (chl a), chlorophyll b (chl b), carotenoids (carot), total chlorophyll (tot chl), chlorophyll a chlorophyll b ratio (Chla/Chlb) and total chlorophyll carotenoids ratio (tot chl/carot) of durum wheat.
The pigment content significantly decreased in all cultivars with increasing water stress. A decrease in chlorophyll b was noticed following cessation of watering, and values were about 4.69 μg.g-1Fw and 3.14 μg.g-1Fw respectively in 30 and 60% PC. With declining soil moisture, at anthesis, chlorophyll b decreased to reach 60.9% and 41.6% respectively at 30 and 60% PC. Chlorophyll a decrease from 17.01 μg.g-1Fw in control to 11.22 μg.g-1Fw in 60% PC and 8.11 μg.g-1fw in 30% PC (Table 2).
Carotenoids content was about 6.25 μg.g-1Fw in control which decreases to 5.26 μg.g-1Fw and 3.85 μg.g-1Fw in 60 and 30% PC respectively. Total chlorophyll carotenoids ratio was higher under control compared with water stress. Chlorophyll a Chlorophyll b ratio was higher under water stress compared to the control conditions. The genotype by treatment interaction was not significant for all studied traits.
The effect of water regimes and organs on waxes composition: Statistical analyzes concerning the composition and the amount of different constituents showed that the differences are highly significant between the organs (flag leaf and spike) (p<0.001), they are also highly significant between water regimes (p<0.001) (Table 3).
T | O | Acet ates µg cm-2 | Hydr o carb ons µg cm-2 | Fatt y acid s µg cm-2 | Alka nes µg.cm-2 | Bi cyclic al Alkan es µg.cm-2 | Alce nes µg cm-2 | Alco hols µg cm2 | Alde hyde s µg cm-2 | Keto nes µg.c m-2 | Chole sterol s µg cm-2 | Est ers µg cm-2 | Eth ers µg cm-2 | Hete ro cyca ls µg cm-2 | Tri terp enes µg cm-2 | Tot al wax es µg.cm-2 |
---|---|---|---|---|---|---|---|---|---|---|---|---|---|---|---|---|
100 % | leaf | 1508a | 3622a | 1156a | 19.3 3a | 132a | 0 | 1.66a | 5.34a | 264a | 0 | 164a | 31. 80a | 0 | 0 | 690 4a |
spike | 0 | 5910a | 331 9a | 0 | 610a | 3.00a | 10.88 | 24.23a | 2475a | 28.49a | 138 0a | 94. 62a | 29.34a | 97.13a | 139 81a | |
means | 754 | 4766 | 223 7 | 9.6 | 371 | 1.5 | 6.27 | 14.78 | 1369 | 14.24 | 772 | 63. 21 | 14.67 | 48.57 | 104 42 | |
60 % | leaf | 2904ab | 5775b | 179 2a | 91.70a | 197a | 0 | 2.45a | 7.29a | 271a | 0 | 209a | 48. 22ab | 0 | 0 | 113 72ab |
spike | 0 | 8312ab | 637 7a | 0 | 610a | 5.05ab | 8.09a | 27.44a | 4376ab | 34.14a | 1861a | 195ab | 74.02a | 199ab | 220 05b | |
means | 1452 | 7043 | 408 4 | 45.85 | 404 | 2.52 | 5.27 | 17.36 | 2324 | 17.07 | 103 5 | 122 | 37 | 99.3 7 | 166 89 | |
30 % | leaf | 4980b | 9756b | 290 1a | 202b | 338a | 0 | 5.29a | 12.55a | 501a | 0 | 415a | 8427b | 0 | 0 | 191 97b |
spike | 0 | 12969b | 706 1a | 0 | 1178a | 6.03b | 9.12a | 41.71a | 5543b | 93.09b | 2903a | 270b | 137.06a | 282b | 30493c | |
means | 2490 | 11362 | 498 1 | 101 | 758 | 3.02 | 7.2 | 27.13 | 3022 | 46.54 | 1659 | 177 | 68.53 | 141 | 24845 | |
An ova | O O | 0 | 0 | 0 | 0 | 0.001 | 0 | 0 | 0 | 0 | 0 | 0 | 0 | 0 | 0 | 0 |
T | 0 | 0 | 0 | 0 | 0 | 0 | 0 | 0 | 0 | 0 | 0 | 0 | 0 | 0 | 0 | |
O × T | 0 | 0 | ns | 0 | Ns | 0 | ns | ns | 0 | 0 | 0 | ns | 0 | 0 | 0.001 |
Table 3. Effect of different levels of water regimes and organ on waxes composition and total waxes amount of Chen’s genotype of durum wheat.
The chemical composition of waxes varied among organs (flag leaf, spike) (Fig. 1). The average of total flag leaf wax amount was 12491μg.cm-2 and of spike was 17965 μg.cm-2.
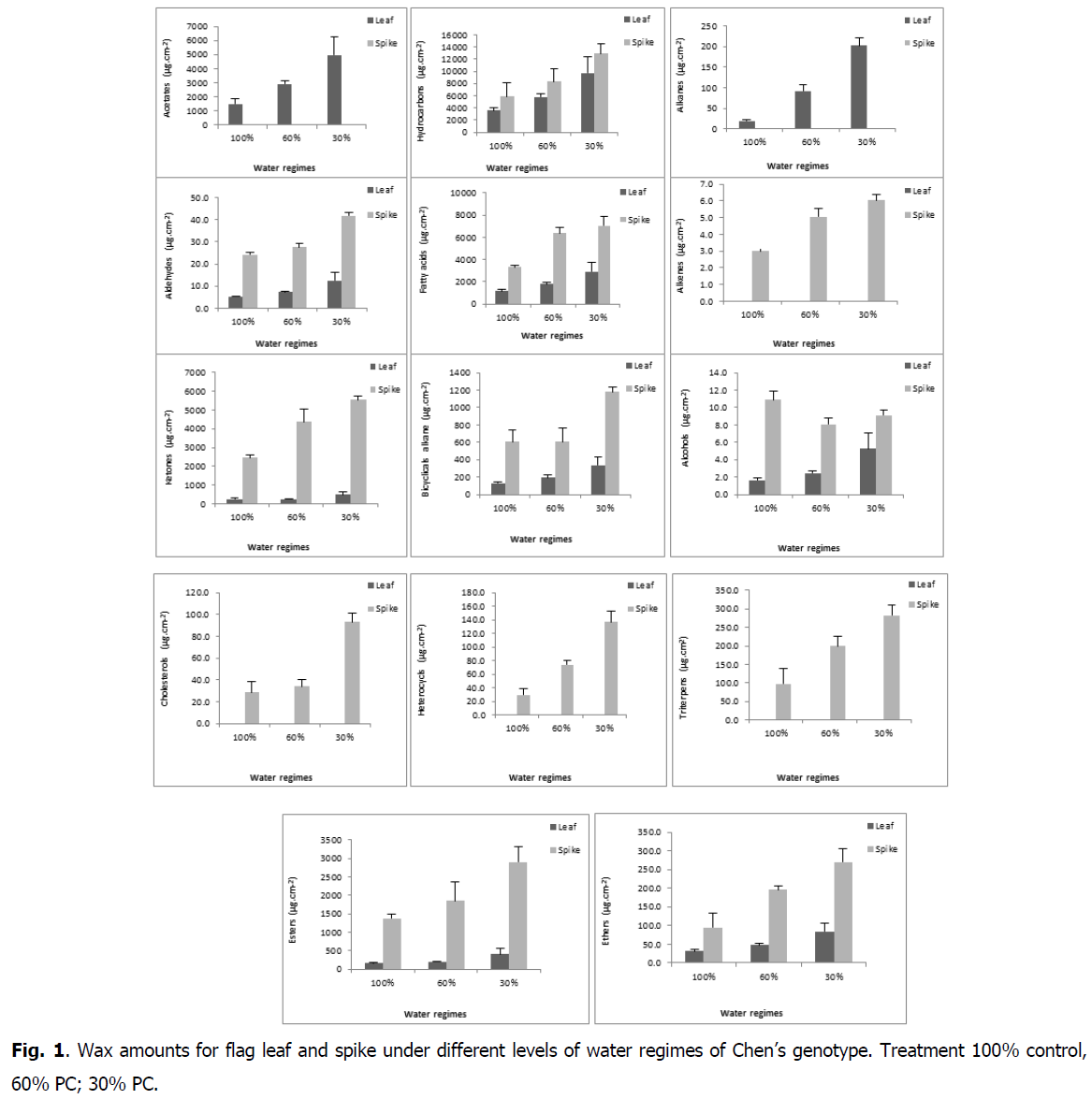
Fig 1: Wax amounts for flag leaf and spike under different levels of water regimes of Chen’s genotype. Treatment 100% control, 60% PC; 30% PC.
The increase in water regimes significantly affected waxes of the both organs (flag leaf and spike), which increased for flag leaf from 6904 μg.cm-2 (control) to 11372 μg.cm-2 and 19197 μg.cm-2 under 60 and 30% PC respectively and for spike from 13981 μg.cm-2 under control to 22005 μg.cm-2 and 30493 μg.cm-2 under 60 and 30% PC, respectively (Fig. 2 and 3).
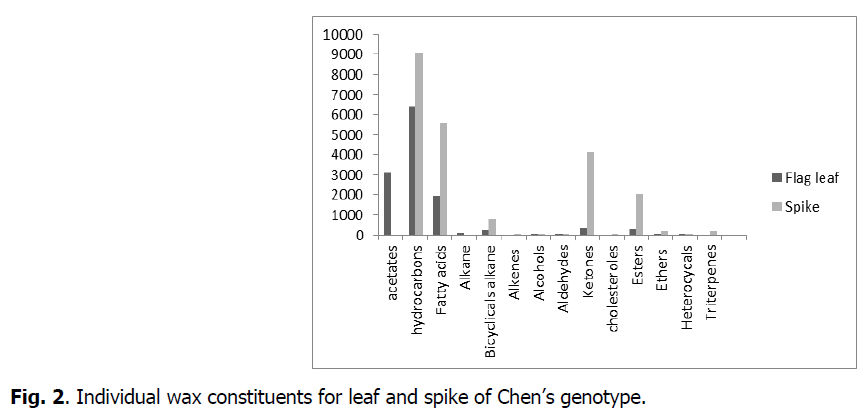
Fig 2: Individual wax constituents for leaf and spike of Chen’s genotype.
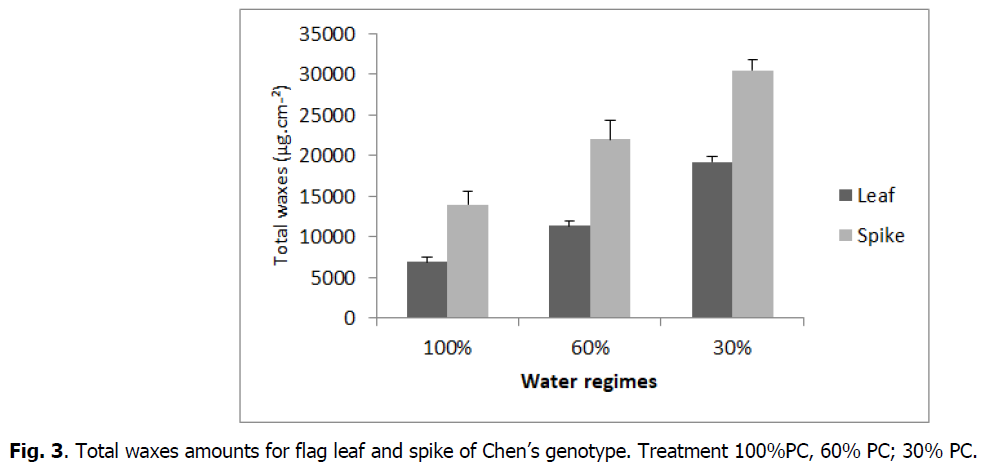
Fig 3: Total waxes amounts for flag leaf and spike of Chen’s genotype. Treatment 100%PC, 60% PC; 30% PC.
Effect of water regimes and genotypes on shoot dry weight, SLDW, different stable isotopes signatures and root shoot ratio (RD/SD): Significant genotypic effect existed for all traits included in Table 4. Water regime significantly affected all studied traits except δ15N, N% and SLDW. Water stress increase significantly root shoot ratio (RD/SD) by 0.41 under (30% PC) and 0.29 under (60% PC) compared with WW conditions. Water stress caused significant losses in shoot dry weight, which decrease from 22.70 g under control to 17.17 g and 15.75 g under 60 and 30% PC respectively. Water stress increase significantly δ13C in all genotypes under water stress conditions in comparison to control conditions. δ13C increase from -30.02‰ under control to -29.47‰ and -28.06‰ under 30 and 60% PC respectively. The δ15N significantly decreased in all cultivars with increasing water limitation, which passed from 6.69‰ under control to 5.53‰ and 5.34‰ under 30 and 60% PC respectively (Fig. 4). Water stress decrease specific leaf dry weight (SLDW) from 4.93 g.cm-2 under control to 4.21 under 60% PC and 3.44 g.cm-2 under 30% PC. Nevertheless, interactions between genotypes and water regimes (G x T) were not significant for all studied traits.
T | G | SDW (g) | Ratio RD/SD | SLDW (g.cm-2) | δ13C (‰) | C% | δ15N (‰) | N% |
---|---|---|---|---|---|---|---|---|
100% | Chen's | 21.16a | 0.186a | 5.027a | -31.07a | 37.30a | 7.24a | 1.87b |
Acsad | 27.27a | 0.218ab | 5.317a | -31.31a | 38.93a | 6.77a | 1.97a | |
Waha | 22.89a | 0.261ab | 4.355a | -28.88a | 38.33a | 6.36a | 1.80a | |
Bidi 17 | 19.53a | 0.342ab | 4.756a | -29.41a | 37.03a | 6.19a | 1.43a | |
Hedba | 22.36a | 0.457b | 5.240a | -29.43a | 38.17a | 6.90a | 1.67a | |
Means | 22.7 | 0.293 | 4.939 | -30.02 | 37.96 | 6.69 | 1.75 | |
60% | Chen's | 13.53a | 0.176a | 4.338a | -31.11a | 39.40ab | 4.72a | 1.73ab |
Acsad | 18.13ab | 0.197a | 4.470a | -30.54ab | 39.63a | 5.10ab | 1.83a | |
Waha | 21.49b | 0.223a | 4.470a | -29.35bc | 39.43a | 5.28ab | 1.80a | |
Bidi 17 | 18.02ab | 0.397b | 3.854a | -28.13c | 39.53a | 6.50c | 1.67a | |
Hedba | 15.30a | 0.483b | 3.958a | -28.21c | 39.30ab | 6.04bc | 1.67a | |
Means | 17.17 | 0.295 | 4.218 | -29.47 | 39.46 | 5.53 | 1.74 | |
30% | Chen's | 11.72a | 0.276a | 3.253a | -29.19a | 39.93b | 5.19a | 1.50a |
Acsad | 20.85c | 0.404ab | 3.364a | -29.00a | 40.56a | 4.82a | 1.97a | |
Waha | 13.71ab | 0.313a | 3.306a | -27.37a | 39.93a | 4.58a | 2.03a | |
Bidi 17 | 19.00bc | 0.449ab | 3.912a | -28.02a | 39.83a | 6.07a | 1.63a | |
Hedba | 14.66ab | 0.640b | 3.391a | -27.06a | 40.40b | 5.77a | 1.80a | |
Means | 15.75 | 0.417 | 3.445 | -28.06 | 40.13 | 5.34 | 1.78 | |
ANOVA | G | 0.001 | 0 | ns | 0 | 0.011 | ns | ns |
T | 0 | 0.008 | 0 | 0 | ns | 0 | 0.001 | |
G × T | ns | ns | ns | ns | ns | ns | ns |
Table 4. Effect of different levels of water regime and genotype on shoot dry weight (SDW) root shoot ratio, SLDW, stable carbon isotope composition (δ13C) and stable nitrogen isotope composition (δ15N) of durum wheat.
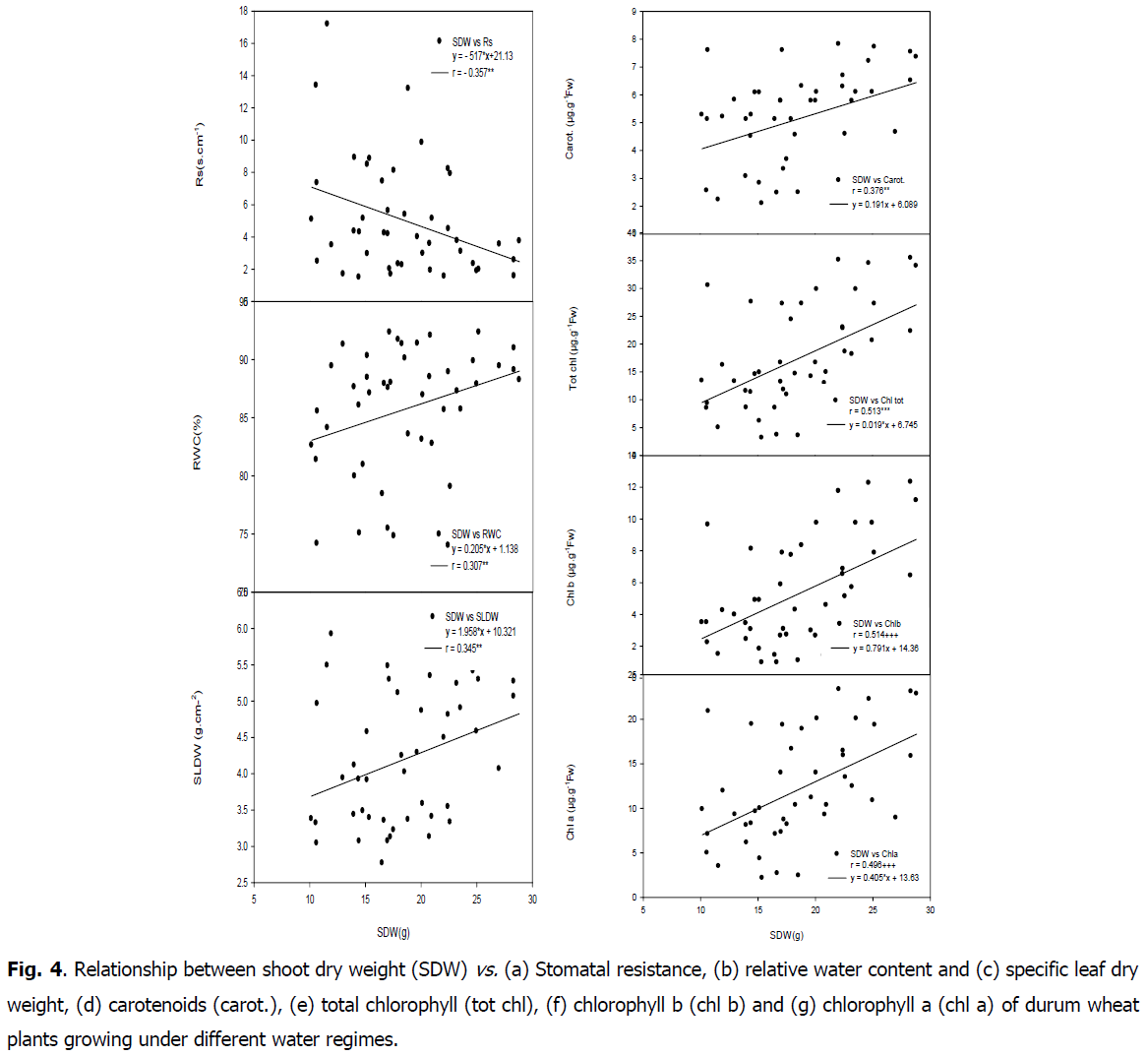
Fig 4: Relationship between shoot dry weight (SDW) vs. (a) Stomatal resistance, (b) relative water content and (c) specific leaf dry weight, (d) carotenoids (carot.), (e) total chlorophyll (tot chl), (f) chlorophyll b (chl b) and (g) chlorophyll a (chl a) of durum wheat plants growing under different water regimes.
Relationships between shoot dry weight and physiological parameters
Positive correlation was found between shoot dry weight and RWC (r=0.307, P<0.05) as well as with SLDW (r=0.345, P<0.05). A negative and highly significant relationship was found between RWC and soluble sugar (r=-0.470, P<0.01) as well as with ratio DR/DS (r=-0.541, P<0.01). Shoot dry weight was negatively correlated with carbon isotope composition (r=-0.317, P<0.05), whereas it was positively correlated with nitrogen isotope composition (r=0.454, P<0.01). Positive correlations were found between shoot dry weight and leaf chlorophyll content (chla r=0.496, P<0.01; chlb r=0.514, P<0.01; carot r=0.376, P<0.05; tot chl r=0.513, P<0.01; tot chl/carot r=0.448, P<0.01). A negative relationship was found between shoot dry weight and stomatal resistance (r=-0.357, P<0.05) (Table 5).
SLDW | chl a | chl b | carot | tot chl | totchl/carot | RS | δ13C | δ15N | RWC | |
---|---|---|---|---|---|---|---|---|---|---|
SDW | 0.345* | 0.496** | 0.514*** | 0.376** | 0.513*** | 0.336** | -0.357** | -0.317** | 0.454** | 0.454** |
δ13C | 0.366* | -0.375* | -0.411** | -0.334** | -0.397** | -0.336** | 0.373** | |||
RS | -0.256ns | -0.389* | -0.403** | -0.396** | -0.403** | -0.317** |
Table 5. Correlation coefficient between shoot dry weight (SDW), δ13C and stomatal resistance and physiological parameters.
Relationship between carbon isotope composition, stomatal resistance and chlorophyll content: A negative correlations were showed between carbon isotope composition and SLDW (r=-0.366, P<0.05) as well as with chlorophyll content (chla r=-0.375, P<0.05; chl b r=-0.411, P<0.01; carot r=-0.334, P<0.05; tot chl r=-0.397, P<0.05 and ratio tot chl/carot r=-0.336, P<0.05) (Fig. 5 and 6).
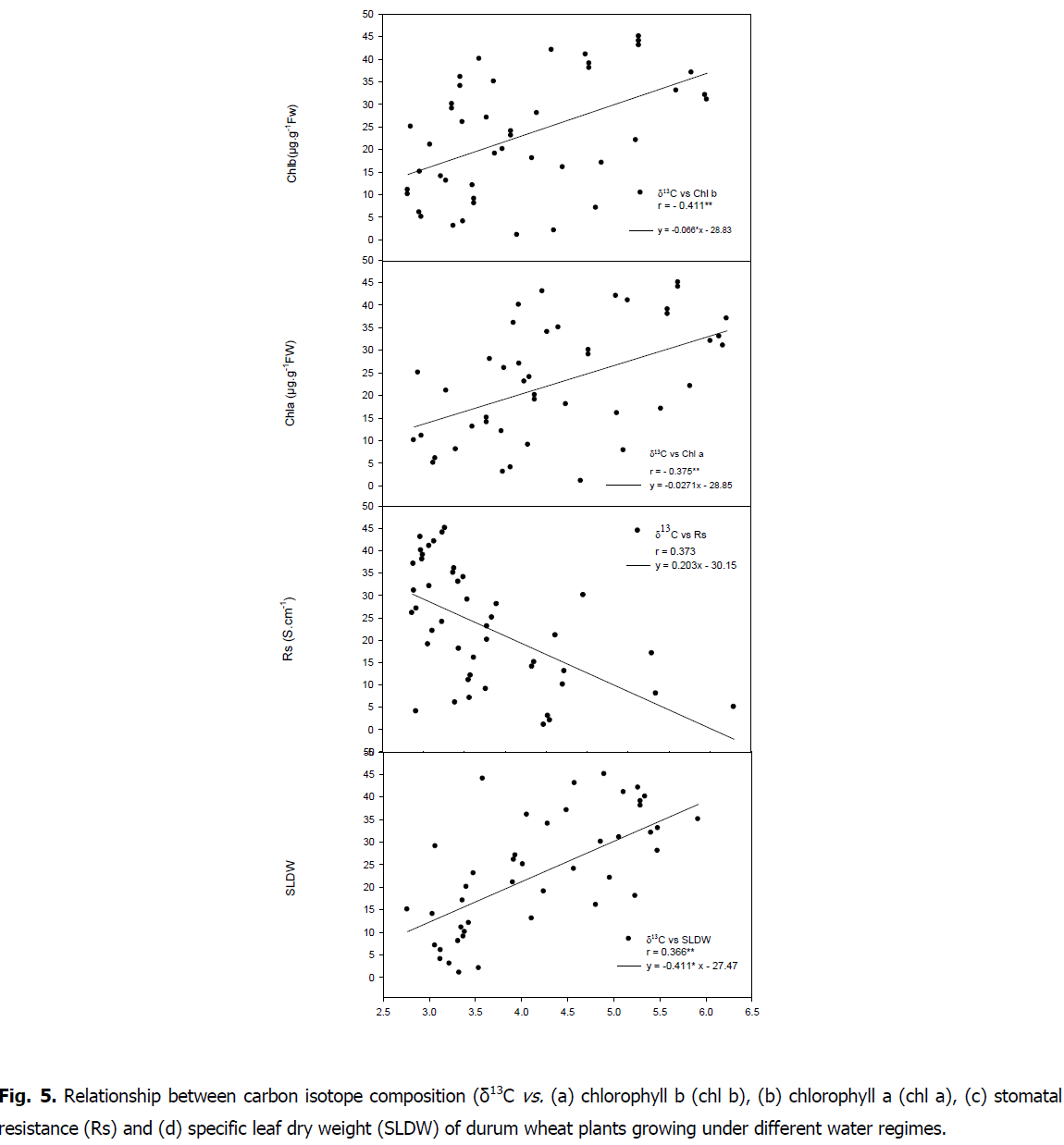
Fig 5: Relationship between carbon isotope composition (δ13C vs. (a) chlorophyll b (chl b), (b) chlorophyll a (chl a), (c) stomatal resistance (Rs) and (d) specific leaf dry weight (SLDW) of durum wheat plants growing under different water regimes.
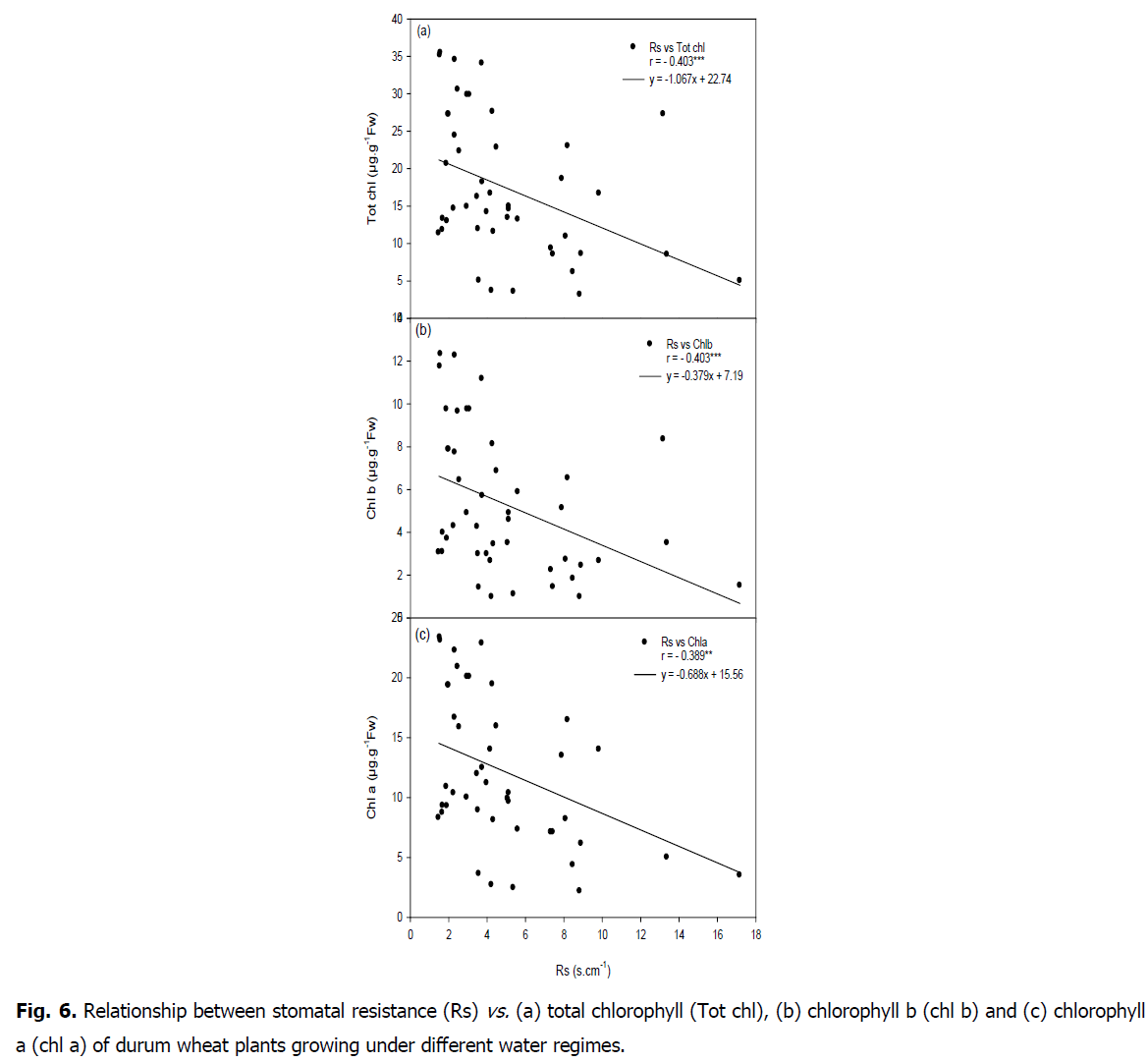
Fig 6: Relationship between stomatal resistance (Rs) vs. (a) total chlorophyll (Tot chl), (b) chlorophyll b (chl b) and (c) chlorophyll a (chl a) of durum wheat plants growing under different water regimes.
A positive correlation was found between carbon isotope composition and stomatal resistance (r=0.373, P<0.05). A negative relationship was showed between stomatal resistance and chlorophyll content (chla r=-0.389, P<0.01; chl b r=-0.403, P<0.01; carot r=-0.396, P<0.01; tot chl r=-0.403, P<0.01; ratio totchl/carot r=-0.317, P<0.05). A positive correlation between SLDW and RWL (r=0.625, P<0.01) was found.
Wax was inversely correlated with RWC (r=-0.70, P<0.001) and transpiration (r=-0.63, P<0.001).
Discussion
Effect of water stress on plant growth has been discussed extensively (Hsiao, 1973; Lu et al., 1998; Araus et al., 2003). Although water stress affects most of the functions of plant growth, this effect depends on the level of water stress, the length of time to which the plant is subjected to water stress and the genotype of plant species (Lu et Neumann, 1998). It is clear from the results obtained in this study, that different levels of water stress have affected the growth of durum wheat cultivars differently, which indicates that the durum wheat cultivars differed in their ability to respond to different levels of water stress. This will help to discover more growth and physiological parameters (RWC, transpiration, stomatal resistance, stable isotope signatures, shoot dry weight, ratio RD/SD, waxes, chlorophyll content, soluble sugar, SLDW) that might be related to water stress sensitivity.
Decreases in shoot dry weight and increase in the ratio RD/SD are well known responses of plants to water stress (Zhang, 1995; Tambussi et al., 2007). Root Shoot Ratio is one of several ratios, which give estimates of the distribution of dry matter between the different plant organs (Wasson et al., 2012; Carvalho et al., 2014). It is a measure of the distribution of dry matter between the root and the shoot systems.
Our results indicated that the water stress led to increase in root shoot ratio, with Acsad and Chen’s the most affected cultivars under the most severe water stress conditions with respectively 85.5% and 48.5% compared with theirs WW plants.
This trend to decrease this ratio has been widely reported (e.g., Abdalla and El-Khoshiban, 2007) as the root was not affected by water stress, and the decrease in the total dry weight is due to the lack of dry weight of shoot. Researchers considered the increase in root growth as an indicator of the ability of plants to withstand water stress, as well as to screen plant cultivars for drought tolerance (Richards et al., 2010).
Under water deficit stress, specific flag leaf dry weight (SLDW) was sharply reduced due a combination of leaf growth reduction. Reduced expansion of younger leaves caused a decrease in the SLDW in stressed plants. The present result is in agreement with the findings in wheat (Tambussi et al., 2007; El Fakhri et al., 2010) and many other herbaceous crop species (e.g., Carvalho et al., 2014).
The relative water content (RWC) is considered as one of the easiest agricultural parameters that can be used to screen for plants drought tolerance. It is a useful indicator of the state of water balance of a plant, essentially because it expresses the absolute amount of water, which the plant requires to reach artificial saturation (Gonzalezet al., 2010; Ganji et al., 2012).
Significant positive correlation was found between RWC and shoot dry weight. This result confirms previous works on durum wheat and bread wheat (Larbi, 2004), showing the effect of water stress on RWC in wheat plants.
Water deficit has exerted a negative effect on RWC, thus in the presence of water stress, the testing genotype lose much more water than under will water conditions. The ability of the plant to survive severe water deficits depends on its ability to restrict water loss through the leaf epidermis after the stomata have attained minimum aperture (Larbi, 2004). These results are the same as reports of Araus et al. (2000) and el Jaafari (2000) and generally are agreement with result of this study. On the other hand, difference in RWC of genotype that are under drought stress may be for this reason that the ability of more absorption of water from soil or ability of stomata to reduce the loss of water is different (Keyvan, 2010). Boutraa et al. (2010) expressed with increase of drought stress of wheat, RWC decrease, in drought stress conditions, the cultivars that are resistant to drought have more RWC. RWC is closely related with cell volume and it may closely reflect the balance between water supply to the leaf and transpiration rate (Araus et al., 2000).
Higher transpiration rates have been reported in leaves from well water than in water stress plants tested genotypes. This difference is due may be to the physical action of water on the cuticle (Clarke and Richards 1989) or to differences in cuticle and leaf hydration. Seo Gonzalez et al. (2011) reviewed literature suggesting that drying of the cuticle reduced residual transpiration rates as water-stress increased. Similar result obtained in wheat (Merah et al., 2000; Kosma et al., 2009; Zhang et al., 2013) and Barley (Gonzalez et al., 2010).
Cuticular transpiration represents the main way of water loss during night under optimal conditions and during noon under drought conditions, when stomata are closed. A lack of a tight closure by stomata, together with the cuticular transpiration, also contribute to the so called residual transpiration (Manzoni et al., 2011; Tian et al., 2013). A low residual transpiration has been proposed as selection trait in wheat breeding for drought resistance (Clarke et al., 1991; Chahbar et al., 2016). Likewise, Schoppach et al., 2012 reported that a low rate of water loss through the plant cuticle is reported to be an important drought survival mechanism and constitutes a substantial proportion of total transpiration in drought-stressed wheat.
A low stomatal resistance (or alternatively a high stomatal conductance) is a good indicator of good water status. Moreover, under water limitation conditions genotypes with comparatively lower stomatal resistance are more resilient to water stress (Motzo et al., 2013). In addition keeping leaf cool via transpiration is a protective mechanism against heat frequently associated to water stress (Tesfaye et al., 2013).
In general, mild water stress is considered to restrict net assimilation rate via its effect on stomatal resistance while under severe drought, reduction of net assimilation rate is ascribed to impairments in biochemical (decrease of net assimilation rate at relatively constant and photochemical reactions (Ninou et al., 2013; Chahbar., et al., 2016).
Carbon isotope composition (δ13C) under water stress increased for all studied genotypes compared with non stress conditions, which agree with previous studies (Ferrio et al. 2005; Cabrera-Bosquet et al. 2009; Araus et al. 2013). High δ13C may also reflect higher stomatal conductance, particularly after anthesis, when soil moisture decreases and water stress becomes stronger (Misra et al., 2010).
SLDW positively related to leaf thickness and/or to compact mesophyll cells both of them reducing CO2 diffusion in the mesophyll and thus increasing carbon isotope composition. Indeed, the carbon isotope composition depends not only on stomatal conductance but also on mesophyll conductance and so on CO2 concentration at chloroplast level (Merah, 2001). This hypothesis is consistent with the positive correlation between SLDW and transpiration and the negatives correlations between the carbon isotope composition, SLDW and chlorophyll content. Similar responses have been reported by Wright et al., 1994. The relation between shoot dry weight and carbon isotope composition was examined and showed a positive relation.
Chlorophyll content was positively correlated to shoot dry weight. The genotype with high shoot dry weight also had high chlorophyll content in well water and water stress conditions. These results agree with the explanation given by Zaharieva et al. (2001). Akhkha et al. (2011) reported that drought tolerance genotypes have high chlorophyll content and high shoot dry weight compared with genotypes under different water stress conditions. Chlorophyll content has been proposed as a screening criterion for wheat tolerance. However, the expected decrease in leaf chlorophyll as a result of water deficit effect was probably offset in our study by an increase in leaf thickness or packing of mesophyll cells as a response to water stress which eventually translated into constant chlorophyll readings (Flexas et al., 2013; Ganji Arjenaki et al., 2012; Chahbar et al., 2016). The decreased level of chlorophyll content is caused by photoinhibition and photo destruction of pigments and pigment-protein complexes and destabilization of photosynthetic membrane both induced by drought (Attia, 2007; Araus et al., 2008, Keyvan, 2010).
The results showed that epicuticular waxes had similar compositions except for certain constituents. Acetates, hydrocarbons, fatty acids, alkanes, bicyclical alkanes, alkenes, alcohols, aldehydes, ketones, cholesterols, esters, ethers, heterocyclicals and triterpenes were the main determined compounds. The results also showed, that some constituents were present in both organs but in varying amounts. Others were present in one organ but not in the other. In leaves, we did not note the presence of alkenes, cholesterols, heterocyclicals and triterpenes as had been the case for spikes. In the spikes, we didn’t note the presence of alkanes and acetates. The chemical composition of waxes varied among organs (leaf or spike) but it still retains its primary role that was waterproofing.
Water stress increased total waxes amount in flag leaf and spike compared with WW plants (Xue et al., 2017). Waxes increase under drought conditions in sorghum (Hwang et al., 2002), wheat (Merah et al. 2001; Koch et al., 2006) pea (Sanchez et al. 2001) and peanut (Samdur et al. 2003; Burow et al. 2008). Waxes are known to improve the efficiency of water use, regulating the quantity of moisture lost via transpiration.
An important function of waxes is to increase the efficiency of stomatal control by reducing water loss after stomatal closure. Indeed, plant survival during severe water deficits depends on the ability to restrict water loss through the leaf epidermis (loss through cuticle plus loss due to incomplete stomata closure) may comprise up to 50% of total transpiration in water-stressed plants during the day and 100% during the night (Araus et al., 2000). Similar responses have been reported for other plants exposed to water deficit, including sesame (kim et al., 2007), Arabidopsis (Kosma et al., 2009) dicotyledonous, and graminaceous species (Shepherd and Wynne Griffiths, 2006; Kim et al., 2007a, 2007b; Kosma et al., 2009; Lu et al., 2009).
Waxes are deposited in different ways and with different structures, forming an interface between the plant aerial organs and the environment (Jenks et al., 2010). Although waxes of all plant species consist of alkanes, primary and secondary alcohols, wax monoesters, aldehydes and free fatty acids, the quantitative distribution differs according to species. Likewise, we observed that durum wheat plants exposed to water deficit possessed greater amounts of total waxes per surface area, with these being explained mainly by an increase in the amount of hydrocarbons and fatty acids.
References
Abdalla, M.M., El-Khoshiban, N.H. (2007). The influence of water stress on growth, relative water content, photosynthetic pigments, some metabolic and hormonal contents of two Triticium aestivum cultivars. Journal of Applied Sciences Research, 3:2062-2074.
Akhkha, A., Boutraa, T., Alhejely, A. (2011). The rates of photosynthesis, chlorophyll content, dark respiration, proline and abscicic acid (ABA) in wheat (Triticum durum) under water deficit conditions. International Journal of Agriculture and Biology, 13:215-221.
Ammar, K., Kronstad, W.E., Morris, C.F. (2000). Breadmaking quality of selected durum wheat genotypes and its relationship with high molecular weight glutenin subunits allelic variation and gluten protein polymeric composition. Cereal Chemistry, 77:230-236.
Qariani, L., El Jaafari, S., Dekkaki, M., Araus, J.L. (2000). Cuticular conductance, water use efficiency and drought tolerance of durum wheat isolines of differing glaucousness. Options Méditerranéennes Série A, séminaires Méditerranéens, 40:315-318.
Araus, J.L., Bort, J., Steduto, P., Villegas, D., Royo, C. (2003). Breeding cereals for Mediterranean conditions: ecophysiological clues for biotechnology application. Annals of Applied Biology, 142:129-141.
Google Scholar, Crossref, Indexed at
Araus, J.L., Cabrera-Bosquet, L., Serret, M.D., Bort, J., Nieto-Taladriz, M.T. (2013). Comparative performance of δ13C, δ18O and δ15N for phenotyping durum wheat adaptation to a dryland environment. Functional Plant Biology, 40:595-608.
Google Scholar, Crossref, Indexed at
Araus, J.L., Febrero, A., Vendrell, P. (1991). Epidermal conductance in different parts of durum wheat grown under Mediterranean conditions: the role of epicuticular waxes and stomata. Plant, Cell and Environment, 14:545-558.
Google Scholar, Crossref, Indexed at
Araus, J.L., Slafer, G.A., Royo, C., Serret, M.D. (2008). Breeding for yield potential and stress adaptation in cereals. Critical Reviews in Plant Science, 27:377-412.
Google Scholar, Crossref, Indexed at
Araus, J.L., Cairns, J.E. (2014). Field high-throughput phenotyping: the new crop breeding frontier. Trends in Plant Science, 19:52-61.
Attia, F. (2007). Effet du stress hydrique sur le comportement écophysiologique et la maturité phénolique de la vigne Vitis vinifera L.: étude de cinq cépages autochtones de Midi-Pyrénées.
Boutraa, T., Akhkha, A., Al-Shoaibi, A.A., Alhejeli, A.M. (2010). Effect of water stress on growth and water use efficiency (WUE) of some wheat cultivars (Triticum durum) grown in Saudi Arabia. Journal of Taibah University for Science, 3:39-48.
Google Scholar, Crossref, Indexed at
Burow, M.D., Starr, J.L., Park, C.H., Simpson, C.E., Paterson, A.H. (2008). Identification of QTLs for resistance to early leaf spot (Cercospora arachidicola S. Hori) in an introgression population of peanut (Arachis hypogaea L.). In Proceedings of the 16th Plant and Animal Genome Conference, 12:16.
Cabrera-Bosquet, L., Albrizio, R., Araus, J.L., Nogués, S. (2009). Photosynthetic capacity of field-grown durum wheat under different N availabilities: A comparative study from leaf to canopy. Environmental and Experimental Botany, 67:145-152.
Carvalho, P., Azam‐Ali, S., Foulkes, M.J. (2014). Quantifying relationships between rooting traits and water uptake under drought in Mediterranean barley and durum wheat. Journal of Integrative Plant Biology, 56:455-469.
Chahbar, S., Belkhodja, M. (2016). Water deficit effects on morpho-physiologicals parameters in durum wheat. Journal of Fundamental and Applied Sciences, 8:1166-1181.
Google Scholar, Crossref, Indexed at
Chahbar, S., Belkhodja, M. (2016). Water deficit effects on osmolyts traits in five durum wheat varieties (Triticum durum). International Journal of Innovation and Applied Studies, 17:757-767.
Clarke, J.M., Richards, R.A., Condon, A.G. (1991). Effect of drought stress on residual transpiration and its relationship with water use of wheat. Canadian Journal of Plant Science, 71:695-702.
Clarke, J.M., Romagosa, I., Jana, S., Srivastava, J.P., McCaig, T.N. (1989). Relationship of excised-leaf water loss rate and yield of durum wheat in diverse environments. Canadian Journal of Plant Science, 69:1075-1081.
Coplen, T.B. (2008). Explanatory glossary of terms used in expression of relative isotope ratios and gas ratios. In IUPAC Recommendations 2008. Triangle Park, NC, USA.
El Fakhri, M., Mahboub, S., Benchekroun, M., Nsarellah, N. (2010). Effet du stress hydrique sur les caractéristiques d'enracinement du blé dur (Triticum Durum. Desf). Nature and Technology, 3:6.
El-Fakhri, M., Nsarellah, N., Mahboub, S., Bidani, A., El-Bouhmadi, K. (2008). Tests morphologiques et biochimiques pour la tolérance au stress hydrique chez.
Farquhar, G.D., von Caemmerer, S.V., Berry, J.A. (1980). A biochemical model of photosynthetic CO2 assimilation in leaves of C3 species. Planta, 149:78-90.
Google Scholar, Crossref, Indexed at
Farquhar, G.D., Ehleringer, J.R., Hubick, K.T. (1989). Carbon isotope discrimination and photosynthesis. Annual Review of Plant Physiology and Plant Molecular Biology, 40:503-537.
Farquhar, G.D., Richards, R.A. (1984). Isotopic composition of plant carbon correlates with water-use efficiency of wheat genotypes. Functional Plant Biology, 11:539-552.
Ferrio, P. (2007). Reconstruction of climatic and crop conditions in the past based on the isotope signature of archaeobotanical remains. Universitat de Lleida.
Flexas, J., Diaz-Espejo, A., Gago, J., Gallé, A., Galmés, J., Gulías, J., Medrano, H. (2014). Photosynthetic limitations in mediterranean plants: a review. Environmental and Experimental Botany, 103:12-23.
Google Scholar, Crossref, Indexed at
Arjenaki, F.G., Jabbari, R., Morshedi, A. (2012). Evaluation of drought stress on relative water content, chlorophyll content and mineral elements of wheat (Triticum aestivum L.) varieties. International Journal of Agriculture and Crop Sciences (IJACS), 4:726-729.
Hwang, K.T., Cuppett, S.L., Weller, C.L., Hanna, M.A. (2002). Properties, composition, and analysis of grain sorghum wax. Journal of the American Oil Chemists' Society, 79:521-527.
Google Scholar, Crossref, Indexed at
Jacobsen, S.E., Jensen, C.R., Liu, F. (2012). Improving crop production in the arid Mediterranean climate. Field Crops Research, 128:34-47.
Javelle, M., Klein-Cosson, C., Vernoud, V., Boltz, V., Maher, C., Timmermans, M., Rogowsky, P.M. (2011). Genome-wide characterization of the HD-ZIP IV transcription factor family in maize: preferential expression in the epidermis. Plant Physiology, 157:790-803.
Google Scholar, Crossref, Indexed at
Jenks, M.A., Ashworth, E.N., Janick, J. (2010). Plant epicuticular waxes: function, production, and genetics. Horticultural Reviews.
Jenks, M.A., Anderson, L., Teusink, R., Williams, M. (2000). Leaf epicuticular waxes of Rosa Apollo Parade and Rosa Charming Parade as effected by plant development, drought, and paclobutrazol treatments. Physiology Plant, 112:62-70.
Karbulková, J., Schreiber, L., Macek, P., Šantrůček, J. (2008). Differences between water permeability of astomatous and stomatous cuticular membranes: effects of air humidity in two species of contrasting drought-resistance strategy. Journal of Experimental Botany, 59:3987-3995.
Google Scholar, Crossref, Indexed at
Kazemi, M., Tahmasbi, A.M., Valizadeh, R., Mesgaran, M.D., Naserian, A.A. (2009). Effect of ensiled barley distillers' grains for Holstein dairy cows. Journal of Animal and Veterinary Advances. 8:807-813.
Keyvan, S. (2010). The effects of drought stress on yield, relative water content, proline, soluble carbohydrates and chlorophyll of bread wheat cultivars. Journal of Animal and Plant Science, 8:1051-1060.
Kim, K.S., Park, S.H., Jenks, M.A. (2007). Changes in leaf cuticular waxes of sesame (Sesamum indicum L.) plants exposed to water deficit. Journal of Plant Physiology, 164:1134-1143.
Google Scholar, Crossref, Indexed at
Koch, K., Barthlott, W., Koch, S., Hommes, A., Wandelt, K., Mamdouh, W., Broekmann, P. (2006). Structural analysis of wheat wax (Triticum aestivum, cv ‘Naturastar’L.): from the molecular level to three dimensional crystals. Planta, 223:258-270.
Google Scholar, Crossref, Indexed at
Kosma, D.K., Bourdenx, B., Bernard, A., Parsons, E.P., Lu, S., Joubès, J., Jenks, M.A. (2009). The impact of water deficiency on leaf cuticle lipids of Arabidopsis. Plant Physiology, 151:1918-1929.
Google Scholar, Crossref, Indexed at
Larbi, A., Mekliche, A. (2004). Relative water content (RWC) and leaf senescence as screening tools for drought tolerance in wheat. Options Méditerranéennes. Série A, Séminaires Méditerranéens, 60:193-196.
Lawson, T., Caemmerer, S.V., Baroli, I. (2010). Photosynthesis and stomatal behaviour. In Progress in Botany, Springer, Berlin, Heidelberg, 72:265-304.
Google Scholar, Crossref, Indexed at
Lichtenthaler, H.K. (1987). [34] Chlorophylls and carotenoids: pigments of photosynthetic biomembranes. In Methods in Enzymology, Academic Press, 148:350-382.
Loss, S.P., Siddique, K.H.M. (1994). Morphological and physiological traits associated with wheat yield increases in Mediterranean environments. Advances in Agronomy, 52:229-276.
Lu, Z., Neumann, P.M. (1999). Water stress inhibits hydraulic conductance and leaf growth in rice seedlings but not the transport of water via mercury-sensitive water channels in the root. Plant Physiology, 120:143-152.
Google Scholar, Crossref, Indexed at
Lü, S., Song, T., Kosma, D.K., Parsons, E.P., Rowland, O., Jenks, M.A. (2009). Arabidopsis CER8 encodes LONG‐CHAIN ACYL‐COA SYNTHETASE 1 (LACS1) that has overlapping functions with LACS2 in plant wax and cutin synthesis. The Plant Journal, 59:553-564.
Google Scholar, Crossref, Indexed at
Mantoan, L.P.B., Rolim de Almeida, L.F., Macedo, A.C., Ferreira, G., Boaro, C.S.F. (2016). Photosynthetic adjustment after rehydration in Annona emarginata. Acta Physiologiae Plantarum, 38:1-11.
Google Scholar, Crossref, Indexed at
Maccaferri, M., Sanguineti, M.C., Corneti, S., Ortega, J.L.A., Salem, M.B., Bort, J., Tuberosa, R. (2008). Quantitative trait loci for grain yield and adaptation of durum wheat (Triticum durum Desf.) across a wide range of water availability. Genetics, 178:489-511.
Google Scholar, Crossref, Indexed at
Manzoni, S., Vico, G., Katul, G., Fay, P.A., Polley, W., Palmroth, S., Porporato, A. (2011). Optimizing stomatal conductance for maximum carbon gain under water stress: a meta‐analysis across plant functional types and climates. Functional Ecology, 25:456-467.
Google Scholar, Crossref, Indexed at
Merah, O. (2001). Potential importance of water status traits for durum wheat improvement under Mediterranean conditions. The Journal of Agricultural Science, 137:139-145.
Google Scholar, Crossref, Indexed at
Merah, O. (2001). Relationships between carbon isotope discrimination and mineral composition in durum wheat. CR Academic Science, Paris, Series III, 324:355-363.
Merah, O., Deléens, E., Souyris, I., Nachit, M., Monneveux, P. (2001). Stability of carbon isotope discrimination and grain yield in durum wheat. Crop Science, 41:677-681.
Google Scholar, Crossref, Indexed at
Misra, S.C., Shinde, S., Geerts, S., Rao, V.S., Monneveux, P. (2010). Can carbon isotope discrimination and ash content predict grain yield and water use efficiency in wheat?. Agricultural Water Management, 97:57-65.
Monneveux, P., Rekika, D., Acevedo, E., Merah, O. (2006). Effect of drought on leaf gas exchange, carbon isotope discrimination, transpiration efficiency and productivity in field grown durum wheat genotypes. Plant Science, 170:867-872.
Google Scholar, Crossref, Indexed at
Motzo, R., Pruneddu, G., Giunta, F. (2013). The role of stomatal conductance for water and radiation use efficiency of durum wheat and triticale in a Mediterranean environment. European Journal of Agronomy, 44:87-97.
Google Scholar, Crossref, Indexed at
Nachit, M.M., Elouafi, I., Pagnotta, A., El Saleh, A.H.M.A.D., Iacono, E., Labhilili, M., Sorrells, M.E. (2001). Molecular linkage map for an intraspecific recombinant inbred population of durum wheat (Triticum turgidum L. var. durum). Theoretical and Applied Genetics, 102:177-186.
Ninou, E., Tsialtas, J.T., Dordas, C.A., Papakosta, D.K. (2013). Effect of irrigation on the relationships between leaf gas exchange related traits and yield in dwarf dry bean grown under Mediterranean conditions. Agricultural Water Management, 116:235-241.
Richards, R.A., Rebetzke, G.J., Watt, M., Condon, A.T., Spielmeyer, W., Dolferus, R. (2010). Breeding for improved water productivity in temperate cereals: phenotyping, quantitative trait loci, markers and the selection environment. Functional Plant Biology, 37:85-97.
Google Scholar, Crossref, Indexed at
Samdur, M.Y., Manivel, P., Jain, V.K., Chikani, B.M., Gor, H.K., Desai, S., Misra, J.B. (2003). Genotypic differences and water‐deficit induced enhancement in epicuticular wax load in peanut. Crop Science, 43:1294-1299.
Google Scholar, Crossref, Indexed at
Sangakkara, U.R., Hartwig, U.A., Nösberger, J. (1996). Soil moisture and potassium affect the performance of symbiotic nitrogen fixation in faba bean and common bean. Plant and Soil, 184:123-130.
Schoppach, R., Sadok, W. (2012). Differential sensitivities of transpiration to evaporative demand and soil water deficit among wheat elite cultivars indicate different strategies for drought tolerance. Environmental and Experimental Botany, 84:1-10.
Google Scholar, Crossref, Indexed at
Shabala, S.N., Lew, R.R. (2002). Turgor regulation in osmotically stressed Arabidopsis epidermal root cells. Direct support for the role of inorganic ion uptake as revealed by concurrent flux and cell turgor measurements. Plant Physiology, 129:290-299.
Google Scholar, Crossref, NCBI
Shangguan, Z.P., Shao, M.A., Dyckmans, J. (2000). Nitrogen nutrition and water stress effects on leaf photosynthetic gas exchange and water use efficiency in winter wheat. Environmental and Experimental Botany, 44:141-149.
Google Scholar, Crossref, Indexed at
Shields, R., Burnett, W. (1960). Determination of protein-bound carbohydrate in serum by modified anthrone method. Analytical Chemistry, 32:885-886.
Sieber, P., Schorderet, M., Ryser, U., Buchala, A., Kolattukudy, P., Métraux, J.P., Nawrath, C. (2000). Transgenic Arabidopsis plants expressing a fungal cutinase show alterations in the structure and properties of the cuticle and postgenital organ fusions. The Plant Cell, 12:721-737.
Google Scholar, Crossref, Indexed at
Tambussi, E.A., Bort, J., Araus, J.L. (2007). Water use efficiency in C3 cereals under Mediterranean conditions: a review of physiological aspects. Annals of Applied Biology, 150:307-321.
Google Scholar, Crossref, Indexed at
Tesfaye, S.G., Ismail, M.R., Ramlan, M.F., Marziah, M., Kausar, H. (2014). Effect of soil drying on rate of stress development, leaf gas exchange and proline accumulation in Robusta coffee (Coffea canephora Pierre Ex Froehner) clones. Experimental Agriculture, 50:458-479.
Tian, F., Gong, J., Zhang, J., Zhang, M., Wang, G., Li, A., Wang, W. (2013). Enhanced stability of thylakoid membrane proteins and antioxidant competence contribute to drought stress resistance in the tasg1 wheat stay-green mutant. Journal of Experimental Botany, 64:1509-1520.
Trethowan, R.M., Reynolds, M. (2007). Drought resistance: genetic approaches for improving productivity under stress. In wheat production in stressed environments. Springer, Dordrecht, pp:289-299.
Vadez, V., Kholova, J., Medina, S., Kakkera, A., Anderberg, H. (2014). Transpiration efficiency: new insights into an old story. Journal of Experimental Botany, 65:6141-6153.
Google Scholar, Crossref, Indexed at
Pirzad, A., Shakiba, M.R., Zehtab-Salmasi, S., Mohammadi, S.A., Darvishzadeh, R., Samadi, A. (2011). Effect of water stress on leaf relative water content, chlorophyll, proline and soluble carbohydrates in Matricaria chamomilla L. Journal of Medicinal Plants Research, 5:2483-2488.
Vico, G., Manzoni, S., Palmroth, S., Katul, G. (2011). Effects of stomatal delays on the economics of leaf gas exchange under intermittent light regimes. New Phytologist, 192:640-652.
Google Scholar, Crossref, Indexed at
Wasson, A.P., Richards, R.A., Chatrath, R., Misra, S.C., Prasad, S.S., Rebetzke, G.J., Watt, M. (2012). Traits and selection strategies to improve root systems and water uptake in water-limited wheat crops. Journal of Experimental Botany, 63:3485-3498.
Google Scholar, Crossref, Indexed at
Wright, G.C., Rao, R.N., Farquhar, G.D. (1994). Water‐use efficiency and carbon isotope discrimination in peanut under water deficit conditions. Crop Science, 34:92-97.
Xue, D., Zhang, X., Lu, X., Chen, G., Chen, Z.H. (2017). Molecular and evolutionary mechanisms of cuticular wax for plant drought tolerance. Frontiers in Plant Science, 8:621.
Google Scholar, Crossref, Indexed at
Yasir, T.A., Min, D., Chen, X., Condon, A.G., Hu, Y.G. (2013). The association of carbon isotope discrimination (Δ) with gas exchange parameters and yield traits in Chinese bread wheat cultivars under two water regimes. Agricultural Water Management, 119:111-120.
Zaefyzadeh, M., Quliyev, R.A., BABAYEVA, S., Abbasov, M.A. (2009). The effect of the interaction between genotypes and drought stress on the superoxide dismutase and chlorophyll content in durum wheat landraces. Turkish Journal of Biology, 33:1-7.
Zaharieva, M., Gaulin, E., Havaux, M., Acevedo, E., Monneveux, P. (2001). Drought and heat responses in the wild wheat relative Aegilops geniculata Roth: potential interest for wheat improvement. Crop Science, 41:1321-1329.
Zhang, Z., Wang, W., Li, W. (2013). Genetic interactions underlying the biosynthesis and inhibition of β-diketones in wheat and their impact on glaucousness and cuticle permeability. PloS One, 8:e54129.
Author Info
S. Chahbar1*, D. Mehdeb2, M. Ouis3 and J.L. Araus42Department of Natural and Life Sciences, College of Agronomy Sciences Mostaganem, Algeria
3Department of Biology Ahmed ZabanaUniversity, Relizane, Algeria
4Section, Barcelona and Agrotecnio (Center of of Plant Physiology, University of Barcelona Research in Agrotechnology), Lleida, Spain
Citation: Chahbar, S., Mehdeb, D., Ouis, M., Araus, J.L. (2022). Carbon and nitrogen isotope composition and epicuticular wax of durum wheat (Triticum durum Desf.) plants exposed to water deficit at anthesis. Ukrainian Journal of Ecology. 12:75-93.
Received: 31-Jul-2022, Manuscript No. UJE-22-70836; , Pre QC No. P-70836; Editor assigned: 02-Aug-2022, Pre QC No. P-70836; Reviewed: 13-Aug-2022, QC No. Q-70836; Revised: 19-Aug-2022, Manuscript No. R-70836; Published: 25-Aug-2022, DOI: 10.15421/2022_387
Copyright: This is an open access article distributed under the terms of the Creative Commons Attribution License, which permits unrestricted use, distribution, and reproduction in any medium, provided the original work is properly cited.