Expert Review - (2021) Volume 0, Issue 0
Climate-smart agricultural practices in Ethiopia for mitigation of greenhouse gas emissions: A review
W. Shiferaw*Abstract
This paper aimed to assess climate-smart agricultural practices in Ethiopia, discuss the contribution of climate-smart agricultural practices for mitigation of greenhouse gas emissions, and examine determinant factors of climate-smart agricultural practices in mitigating greenhouse gas emissions. Conservation agriculture, integrated soil fertility management, agroforestry, crop diversification, and improved livestock feed and feeding practices are among Ethiopia's best climate-smart agricultural practices. Combination of the adoption of climate-smart agricultural practices such as zero-tillage, increased crop diversity, and retaining crop residue on-farm can mitigate increased SOC in non-flooded crops that change in a significant ton of CO2e ha-1 year-1. In addition, a mitigation potential of CH4 in reduced irrigation of paddy rice farms was changed in ton CO2e ha-1 year-1. It was found that productivity-enhancing interventions in the tropics could reduce emission intensity in dairy systems by up to 0.9 t CO2e per milk. Agroforestry practices and the addition of organic fertilizers on the farm increased mitigation potential of 784093 t CO2e and 193050 t CO2e biomass of carbon and SOC per year, respectively. Adoptions of climate-smart agricultural practices are affected by factors such as farming factors, technology inaccessibility, environmental factors, policy design and social expertise, negative attitudes and motivations of farmers, farmers' socio-demographic factors, and farmers' socioeconomic factors. To reverse the situations, preparing targeted climate-smart agricultural practices to areas that are likely to provide the most significant GHG reduction potential and demonstrate these practices to other areas should be encouraged to learn for similar agroecology.
Keywords
Climate, Conservation agriculture, GHG emission, Livestock husbandry, Mitigation.
Introduction
In Ethiopia agriculture is the fundamental economic sector, in which most population relies on its social and economic development (Shiferaw and Datt, 2018). Its contribution was 46.3% of the national GDP and 90% of the foreign exchange earnings of the country (Adem et al., 2018). However, climate variability, change, and related extreme events are adversely affecting agricultural production. Climate changes likely result in increased variability in precipitation and an increase in temperature (Dendir and Simane, 2019). The variability in precipitation and temperature increase is due to increases in greenhouse gas (GHG) emissions into the surrounding atmosphere.
Human activities like agriculture have caused climate change; mainly, small-scale farming contributes to greenhouse gas (GHG) emissions and is a victim of climate change (Abegunde et al., 2019). It is estimated that agriculture and associated land-use changes account for 24% of total global emissions (Niles et al., 2018 cited in Smith et al., 2014). However, excluding forestry and other land uses, agriculture contributes approximately 12% of global GHG emissions (IFAD, 2019; Jantke et al., 2020). Methane (CH4) and nitrous oxide (N2O) are the primary GHGs produced by agricultural activities, comprising about 55% and 45% of emissions from agriculture, respectively (IFAD, 2019). In Ethiopia, annual GHG emissions were estimated at 150 Mt CO2e in 2010, with 50% and 37% of these emissions resulting from the agricultural and forestry sectors, respectively. In addition, livestock production accounted for more than 40% of the emissions in agriculture (FAO, 2016).
Climate-smart agriculture (CSA) is an approach to agriculture that sustainably increases productivity, enhances adaptation, and mitigates emissions of GHG (IFAD, 2019). On the other hand, there are inadequate research findings on climate-smart agricultural (CSA) practices in Ethiopia for the various agroecology, soil type, rainfall pattern, farming system, temperature, and moisture ranges. At all levels, data on climate-smart agricultural (CSA) and conservation agriculture, in particular, are insufficient (FAO, 2016). Moreover, little research has reported the status of climate smart agricultural (CSA) practices in mitigating GHG emissions in Ethiopia. Thus, this review paper aimed to assess climate-smart agricultural (CSA) practices in Ethiopia, take up lessons learned elsewhere or in Ethiopia, discuss the contribution of climate-smart agricultural practices for mitigation of greenhouse gas emissions, and examine determinant factors climate-smart agricultural practices in mitigation of greenhouse gas emissions. Thus, the paper addresses relevant scientific information based on evaluating data collected from different journals, manuals, and various report works.
Climate and agriculture
Agriculture is the primary sector of the Ethiopian economy. Agriculture in Ethiopia includes crops, livestock, forestry, fisheries, apiculture, and other natural resources. It is the most critical sector of the national economy and the primary source of livelihood for 85% of the population (FAO, 2016). However, the production and productivity of agriculture in the country are highly vulnerable to climate variability (Dendir and Simane, 2019). Climate variables such as temperature, reduced rainfall, and increased rainfall variability reduces crop yield and threaten food security in low-income and agriculture-based economies (Gezie, 2019).
The effect of climate change is attributed to the reduction of crop yields and incomes, and the human health of the population engaged in agricultural production is generally expected to be adverse (Komarek et al., 2019). In Ethiopia, climate change-related health problems like mortality and morbidity due to floods and heatwaves, vector-borne diseases, water-borne diseases, meningitis, and air pollution-related respiratory diseases increase (Simane et al., 2017).
Climate change has caused recurrent droughts and famines, flooding, expansion of desertification, loss of wetlands, loss of biodiversity, and water shortage resulting in the decline of agricultural production (Zegeye, 2018). IPCC (2013) depicts that spatial and temporal distribution of water resources become uneven due to global climate change effects. Other evidence indicates that the major impact of climate change on Ethiopia's economy will result from the more frequent occurrence of extreme hydrologic events, which cause losses in both the agricultural and non-agricultural sectors (IFPRI, 2010). As a result, the patterns of climate change data show that rainfall is increasingly erratic with marked seasonal deficits when compared to long term past averages, droughts appear to be increasingly frequent, heavy rainfall events appear to be increasingly frequent, with changes in rainfall patterns, including decreased reliability and less predictability for agricultural activities (USAID, 2015).
Supposing that constant inputs of carbon to soils from vegetation, different estimates predict that expected climate changes, such as temperature, precipitation, and evaporation, will cause a significant change in organic matter turnover and CO2 dynamics (Karmakar et al., 2016). Moreover, Daba et al. (2018) show that soil is complicatedly linked to the climate system through nitrogen, carbon, and hydrologic cycles, and in consequence, change in climate affect soil processes and properties. Climate change also increased soil, water bodies, and air pollutions and consequently impacted agricultural production (Arora, 2019). Soil erosion by wind and water is also likely to increase due to climate change, which removes the most fertile part of topsoil (Brevik, 2013). Therefore, climate change impacts on agriculture have significant consequences on livelihoods, food production, and the overall economy of countries. Climate changes affect particularly those with agriculture-based economies in the developing world like Ethiopia on crop production, livestock productivity, horticultural crops, aquaculture (fish production), and apiculture (Lemi and Hailu, 2019).
Concepts and definitions of terminologies
Climate-smart agriculture (CSA) is a tactic for changing and reorienting agricultural systems to support food security under climate change and reduce GHG emissions from agricultural activities (Abegunde et al., 2019; Komarek et al., 2019). According to Neufeldt et al. (2013), climate-smart agriculture (CSA) consists of several agricultural practices that sustainably increase productivity, improve resource use efficiency, reduce exposure, vulnerability to climate variability, and reduce GHG emissions from agriculture. On the other hand, climate smart agriculture (CSA) is a very widely used term that reflects no climate-specific benefits and it contributes significantly to GHG emissions and at the same time is strongly affected by climate change has initiated the exploration of agricultural methods that are aligned with the maintenance of ecosystems (FAO, 2010; SLM, 2017).
Greenhouse gases (GHGs) emission is the release of gases in carbon dioxide; methane and nitrous oxide are enormously into the atmosphere through these major sources such as deforestation, automobile, agriculture, industry, and organic waste (Arfin et al., 2015). Emissions of greenhouse gases (GHGs) are the most important driver of human-induced climate change (Jantke et al., 2020). Whereas climate change mitigation consists of actions to limit the magnitude or rate of long-term global warming and its related effects. Climate change mitigation generally involves reductions in anthropogenic emissions of greenhouse gasses (GHGs).
Sources of GHG emissions from Agriculture
Ethiopia is one of the world's lowest GHG emissions, ranking 182 of 188 countries on per capita emissions and contributing only 0.27% of global emissions (MOFAN, 2018). Today's per capita emissions of less than 2 t CO2e are modest compared with the more than 10 t per capita on average in the European Union (EU) and more than 20 t per capita in USA and Australia (Zegeye, 2018).
According to Engdaw (2020), except for land use and land cover changes in forestry, Ethiopia has shown increasing emission trends in most sectors. However, land use and change and forestry are the first leading sector that has contributed to the country's largest greenhouse gas emissions (Fig. 1).
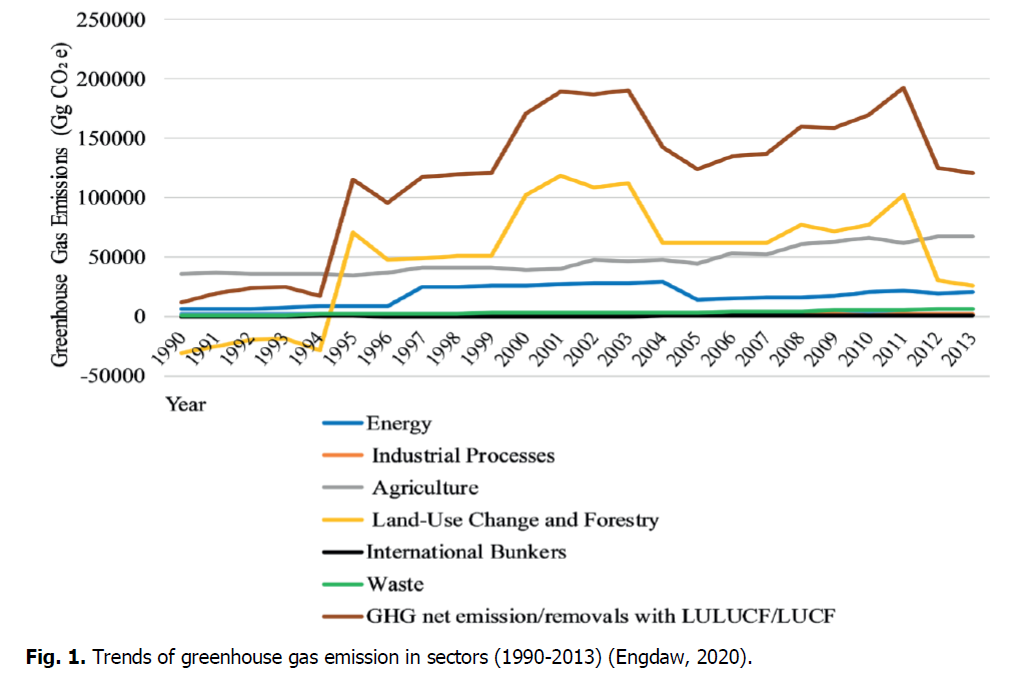
Figure 1: Trends of greenhouse gas emission in sectors (1990-2013) (Engdaw, 2020).
Having an average emission of 50739.7 GgCO2e, land use and land cover change and forestry is the largest sector contributing to greenhouse gas emissions in Ethiopia. The agricultural sector played the second role with an average emission level of 47093.63 GgCO2e. Following the above two sectors, the energy sector that ranked third has contributed an emission of 17670.13 GgCO2e. Other sectors like waste, industrial, and international bunkers have contributed a trivial amount to the country's greenhouse gas emissions, with average greenhouse gas emissions of 3081.21 GgCO2e, 881.21 GgCO2e, and 458.65 GgCO2e, respectively (Engdaw, 2020).
In Ethiopian agriculture in 2012, the significant sources GHG emissions are indicated in Fig. (2). The most significant proportion of emissions results from enteric fermentation (53%), followed by manure left on pasture (37%), both related to livestock production. Nevertheless, the minor emitters in Ethiopian agriculture are rice cultivation, burning crop residues, manure applied on soil, and crop residues (FAO, 2016).
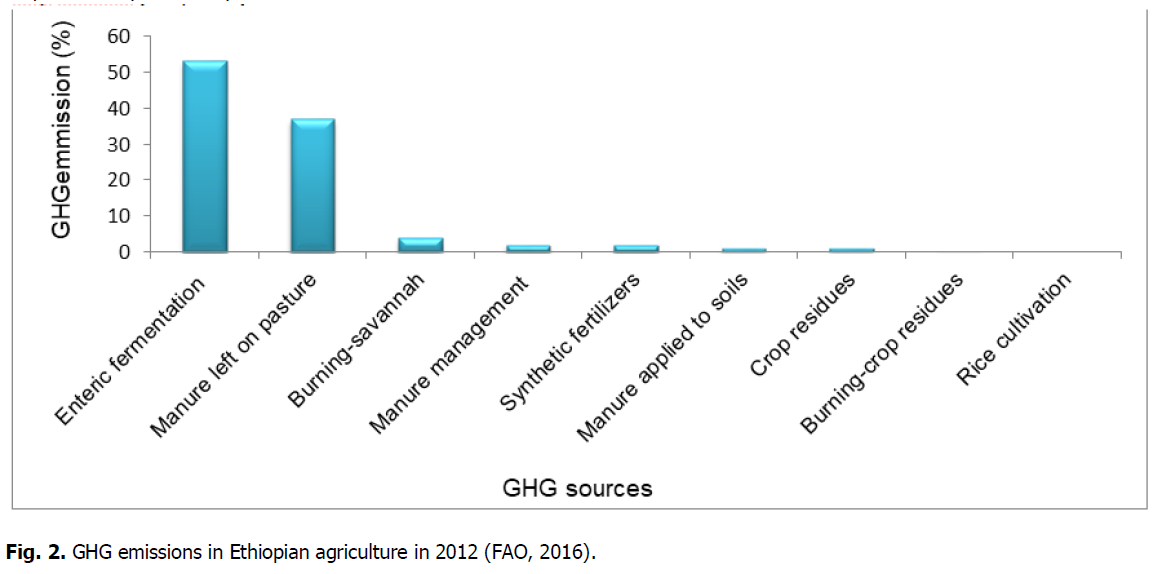
Figure 2: GHG emissions in Ethiopian agriculture in 2012 (FAO, 2016).
Moreover, the share of GHG emissions from the urban production system was 58.4% compared to 22.14% mixed crop-livestock and 18.6% pastoral production systems (Berhe and Bariagabre, 2020). Their study also shows that 83.42% CH4 was emitted from the systems. The rest 16.6%, of the production systems, were constituted by CO2 and N2O GHG gases (Table 1).
GHG emission | PPS | MLPS | UPS | (%) of total |
---|---|---|---|---|
CO2 | 0.79 | 2.94 | 5.75 | 4.18 |
CH4 | 74.77 | 83.93 | 85.97 | 83.42 |
N2O | 24.44 | 13.13 | 8.28 | 12.40 |
% of total | 18.59 | 22.14 | 58.44 | 100 |
Table 1. Contribution of the different modules of the GHG from the three production systems (%) (Berhe and Bariagabre, 2020).
Climate-smart agricultural practices in Ethiopia
Climate innovative agricultural (CSA) practices sustainably increase productivity, enhance resilience, remove GHGs, and enhance national food security and development goals (FAO, 2010). There are different climate-smart agricultural practices in Ethiopia (FAO, 2016). These practices consist of different components and are used for specific purposes in GHG emission mitigation and climate change adaptation (Table 2).
CSA practice | Components | Why it is climate-smart |
---|---|---|
Conservation agriculture | • Reduced tillage • Crop residue management–mulching, intercropping • Crop rotation/intercropping with cereals and legumes |
• Carbon sequestration • Reduce existing emissions • Resilience to dry and hot spells |
Integrated soil fertility management |
• Compost and manure management, including green manuring • Efficient fertilizer application techniques (time, method, amount) |
• Reduced emission of nitrous oxide and CH4 • Improved soil productivity |
Small-scale irrigation | • Year-round cropping • Efficient water utilization |
• Creating carbon sink • Improved yields • Improved food security |
Agroforestry | • Tree-based conservation agriculture • Practiced both traditionally and as improved practice • Farmer-managed natural regeneration |
• Trees store large quantities of CO2 • Can support resilience and improved productivity of agriculture |
Crop diversification | • Popularization of new crops and crop varieties • Pest resistance, high yielding, tolerant to drought, short season |
• Ensuring food security • Resilience to weather variability • Alternative livelihoods and improved incomes |
Improved livestock feed and feeding practices |
• Reduced open grazing/zero grazing • Forage development and rangeland management • Feed improvement • Livestock breed improvement and diversification |
• Improved livestock productivity • GHG reduction • CH4 reduction |
Other | • In situ water conservation/harvesting • Early-warning systems and improved weather information • Support to alternative energy fuel-efficient stoves, biofuels • Crop and livestock insurance • Livelihoods diversification (apiculture, aquaculture) • Post-harvest technologies (agro-processing, storage) |
• Resilience of agriculture • Improved incomes • Reduced emissions • Reduced deforestation • Reduced climate risk |
Table 2. Summary of some standard CSA practices in Ethiopia (FAO, 2016).
Various types of traditional climate-smart agricultural (CSA) practices have also been implemented and adopted in Ethiopia. Such practices include the Derashe traditional conservation agriculture, Konso cultural landscape, and traditional agroforestry in Gedeo Zone, East Shewa Zone, East Wollega Zone, and West Gojam Zone, Hararghe highland traditional soil and water conservation, Hararghe cattle fattening, and Hararghe small-scale traditional irrigation in eastern Ethiopia, and Ankober manure management in the Northern parts of the country (FAO, 2016).
Mitigation potential of climate-smart agricultural practices for GHG emissions
Agriculture can play an essential role in climate change mitigation while increasing food security and rural poverty reductions (FAO, 2012). Widespread adoption of climate-smart agriculture (CSA) has the potential to mitigate significantly agricultural greenhouse gas (GHG) emissions by increasing soil organic carbon (SOC) stocks and decreasing nitrous oxide (N2O) emissions (McNunn et al., 2020). According to IFAD (2019), combination of adopting climate-smart agricultural (CSA) practices such as no tillage, increased crop diversity, and retaining crop residue on the farm has a mitigation potential/or increased SOC in non-flooded crops that change in a significant ton of CO2e ha-1 year-1. In addition, a mitigation potential of CH4 in reduced irrigation of paddy rice farms was also changed in ton CO2e ha-1 year-1 (Fig. 3).
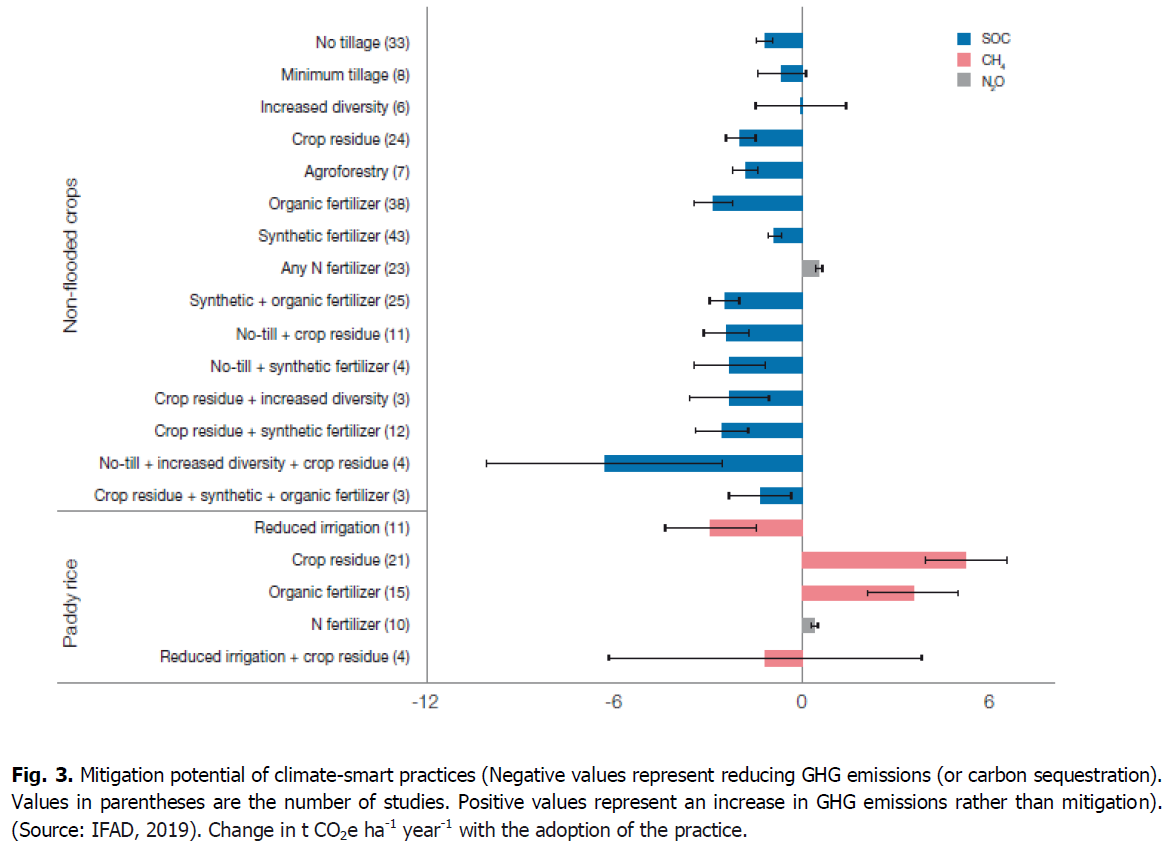
Figure 3: Mitigation potential of climate-smart practices (Negative values represent reducing GHG emissions (or carbon sequestration). Values in parentheses are the number of studies. Positive values represent an increase in GHG emissions rather than mitigation). (Source: IFAD, 2019). Change in t CO2e ha-1 year-1 with the adoption of the practice.
Mitigation practices that increase animal productivity generally also increase emissions but decrease CH4 per unit of animal product (emission intensity). For instance, Thornton and Herrero (2010) found that productivity-enhancing interventions in the tropics could reduce emission intensity in dairy systems by up to 0.9 t CO2e per milk. IFAD (2019) also depicts agroforestry practices, and the addition of organic fertilizers on the farm increased mitigation potential of 784093 t CO2e and 193050 t CO2e-biomass of carbon and SOC per year, respectively (Fig. 4).
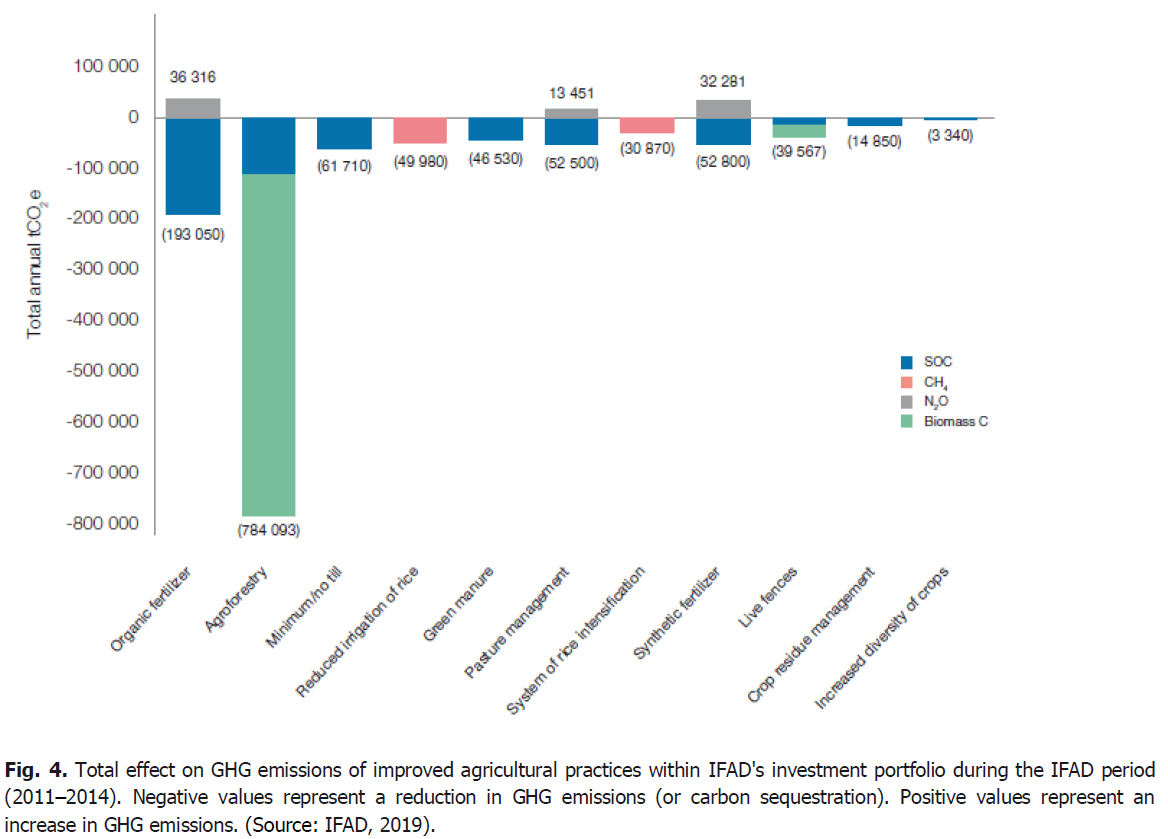
Figure 4: Total effect on GHG emissions of improved agricultural practices within IFAD's investment portfolio during the IFAD period (2011–2014). Negative values represent a reduction in GHG emissions (or carbon sequestration). Positive values represent an increase in GHG emissions. (Source: IFAD, 2019).
Determinant of climate-smart agricultural practices in mitigation of GHG emissions
One of the major strategies of adoption and diffusion of various climate-smart agricultural (CSA) practices for improving crop and livestock production and farmer income while reducing greenhouse gas emissions. However, adoptions of climate-smart agricultural (CSA) practices are affected by different factors. These could be due to farming factors (farm size), technology inaccessibility, environmental factors, policy design and social expertise, negative attitudes and motivations of farmers, farmers' socio-demographic factors, and farmers' socioeconomic factors (Teklewold et al., 2019). The education status of the farmers and access to extension and weather information can also influence the likelihood of adopting these practices (Issahaku and Abdulai, 2019).
Diallo et al. (2019) show that adopting various climate-smart agricultural (CSA) strategies were significantly influenced by the socioeconomic, infestation of pests, credit access, institutional and climate-related factors. Moreover, several factors, including household characteristics, plot characteristics, market access, and significant climate risks, affect the probability and level of adoption of the various climate-smart agricultural (CSA) practices (Aryal et al., 2018).
Conclusions
In Ethiopia, several traditional and adopted climates smart agricultural (CSA) practices carried out by farmers in different agroecology. However, little research information is available for different stakeholders for broader scale-out and scale-up of the technologies. Among the climate-smart agricultural (CSA) practices, agroforestry, conservation agriculture, intensive livestock husbandry, integrated soil fertility management, area closure for restoration of wetlands and degraded lands, participatory forest management practices are the best practices that sustain the ecosystem services, mitigation of greenhouse gases (GHGs), and ultimately improve incomes of households and alleviate poverty.
Combinations of the best-adopted climate-smart agriculture practices have high potential in the mitigation of greenhouse gases. Integration conservation tillage practices with crop diversity on the farmlands play a significant role in reducing GHG emissions. Moreover, enhancing the productivity of livestock could also reduce the intensity of GHG emissions into the atmosphere. However, different adoptions of climate-smart agricultural practices are affected by different factors that have implications for the continuous emissions of GHGs.
To reverse the situations of climate change, so many adaptation options should be designed. For instance, by adopting climate-smart agricultural (CSA) technologies, farmers are encouraged to join community farmer groups to enhance extension service provision through these groups. For extension services, farmers using the best traditional and adopted climate smart agricultural (CSA) practices which should be clustered for agricultural production activities and mitigation of GHG on their farm plots. These farmers can be used as model farmers for agriculture and natural resources management works. Preparation of targeted climate-smart agricultural (CSA) practices to areas that are likely to be learned in provide the most significant GHG reduction potential and demonstrate the practices to other areas should be encouraged to be learn similar agroecology. In addition, further multidisciplinary researches on climate-smart agricultural (CSA) practices should also carried out to select the best technologies at different stakeholder levels in the country.
Acknowledgment
The author thanks Arba Minch University for supporting the document to be prepared.
References
Abegunde, V.O., Sibanda, M., Obi, A. (2020). Determinants of the adoption of climate-smart agricultural practices by small-scale farming households in King Cetshwayo District Municipality, South Africa. Sustainability, 12:195.
Adem, M., Tadele, E., Mossie, H., Ayenalem, M. (2018). Income diversification and food security situation in Ethiopia: A review study. Cogent Food & Agriculture, 4:1513354.
Arfin, T., Aquatar, M.O., Waghmare, S.S. (2015). Mitigation strategies to greenhouse gas emission control: a database for emission factors. IJSRD, 3:908-917.
Arora, N.K. (2019). Impact of climate change on agriculture production and its sustainable solutions.
Aryal, J.P., Rahut, D.B., Maharjan, S., Erenstein, O. (2018). Factors affecting the adoption of multiple climate-smart agricultural practices in the Indo-Gangetic Plains of India. In Natural Resources Forum, 42:141-158.
Berhe, A., Bariagabre, S.A., Balehegn, M. (2020). Estimation of greenhouse gas emissions from three livestock production systems in Ethiopia. International Journal of Climate Change Strategies and Management.
Brevik, E.C. (2013). The potential impact of climate change on soil properties and processes and corresponding influence on food security. Agriculture, 3:398-417.
Daba, H.M., Bazi, Z., Belay, A. (2018). Effects of Climate Change on Soil and Water Resources: A Review. Journal of Environment and Earth Science, 8:71-80.
Dendir, Z., Simane, B. (2019). Livelihood vulnerability to climate variability and change in different agroecological zones of Gurage Administrative Zone, Ethiopia. Progress in Disaster Science, 3:100035.
Diallo, M., Aman, N., Adzawla, W. (2019). Factors influencing the adoption of climate smart agriculture by farmers in Ségou region in Mali. In Proceedings of the Conference on Climate Change and Food Security in West, Africa Dakar, Senegal, pp:17-18.
Engdaw, B.D. (2020). Assessment of the trends of greenhouse gas emission in Ethiopia. Geography, Environment, Sustainability, 13:135-146.
FAO. (2010). "climate-smart" agriculture: policies, practices and financing for food security, adaptation and mitigation. Paper prepared for Hague Conference on Agriculture, Food Security and Climate Change.
Bockel, L., Tinlot, M., Branca, G. (2012). The Carbon Footprint of the Agricultural Growth Project (AGP) in Ethiopia. FAO.
Jirata, M., Grey, S., Kilawe, E. (2016). Ethiopia climate-smart agriculture scoping study. FAO: Addis Ababa, Ethiopia.
Gezie, M. (2019). Farmer's response to climate change and variability in Ethiopia: A review. Cogent Food & Agriculture, 5:1613770.
International Fund for Agricultural Development (IFAD). (2019). Climate change mitigation potential of agricultural practices supported by IFAD investments: An ex ante analysis.
IPCC. (2013). Climate Change 2013: the Physical Science Basis. Contribution of Working Group I to the Fifth Assessment Report of the Inter-governmental Panel on Climate Change. Cambridge University Press, Cambridge, UK.
Issahaku, G., Abdulai, A. (2020). Adoption of climate-smart practices and its impact on farm performance and risk exposure among smallholder farmers in Ghana. Australian Journal of Agricultural and Resource Economics, 64:396-420.
Jantke, K., Hartmann, M.J., Rasche, L., Blanz, B., Schneider, U.A. (2020). Agricultural greenhouse gas emissions: knowledge and positions of German farmers. Land, 9:130.
Das, I., Dutta, D., Rakshit, A. (2016). Potential Effects of Climate Change on Soil Properties: A Review.
Komarek, M.A., Thurlow, J., Koo, J., Pinto, D.A. (2019). Economy wide effects of climate-smart agriculture in Ethiopia. Agricultural Economics, 50:765-778.
Lemi, T., Hailu, F. (2019). Effects of climate change variability on agricultural productivity. International Journal Environmental Science Natural Resoures, 17:14-20.
McNunn, G., Karlen, L.D., Salas, W., Rice, W.C., Mueller, S., Jr, MD., Seale, W.J. (2020). Climate smart agriculture opportunities for mitigating soil greenhouse gas emissions across the U.S. Corn-Belt. Journal of Cleaner Production, 268:122240.
Ministry of Foreign Affairs of the Netherlands (MOFAN). (2018). Climate change profile Ethiopia.
Neufeldt, H., Jahn, M., Campbell, B.M., Beddington, J.R., DeClerck, F., De Pinto, A., Zougmoré, R. (2013). Beyond climate-smart agriculture: toward safe operating spaces for global food systems. Agriculture & Food Security, 2:1-6.
Niles, M.T., Ahuja, R., Barker, T., Esquivel, J., Gutterman, S., Heller, M.C., Vermeulen, S. (2018). Climate change mitigation beyond agriculture: a review of food system opportunities and implications. Renewable Agriculture and Food Systems, 33:297-308.
Gemeda, W.S., Datt, S.P. (2018). Characterization of soils under major land uses in Chencha District, South Ethiopia. Journal of Soil Science and Environmental Management, 9:127-137.
Simane, B., Beyene, H., Deressa, W., Kumie, A., Berhane, K., Samet, J. (2016). Review of climate change and health in Ethiopia: status and gap analysis. Ethiopian Journal of Health Development, 30:28-41.
Sustainable Land Management (SLM). (2017). Climate Smart Agriculture (CSA) a manual for implementing the sustainable land management program (SLMP).
Teklewold, H., Gebrehiwot, T., Bezabih, M. (2019). Climate smart agricultural practices and gender differentiated nutrition outcome: An empirical evidence from Ethiopia. World Development, 122:38-53.
You, G.J.Y., Ringler, C. (2010). Hydro-economic modeling of climate change impacts in Ethiopia (No. 960). International Food Policy Research Institute (IFPRI).
Thornton, P.K., Herrero, M. (2010). Potential for reduced methane and carbon dioxide emissions from livestock and pasture management in the tropics. Proceedings of the National Academy of Sciences, 107:19667-19672.
United States Agency for International Development (USAID). (2015). Climate variability and change in Ethiopia: summery of findings in Zermoglio F, Steynor A, Jack C (Ed). Technical reports.
Zegeye, H. (2018). Climate change in Ethiopia: impacts, mitigation and adaptation. International Journal of Research in Environmental Studies, 5:18-35.
Author Info
W. Shiferaw*Citation: Shiferaw W. (2021). Climate-smart agricultural practices in Ethiopia for mitigation of greenhouse gas emissions: a review. Ukrainian Journal of Ecology, 11 (6), 23-30.
Received: 13-Jul-2021 Accepted: 26-Jul-2021 Published: 23-Aug-2021
Copyright: This is an open access article distributed under the terms of the Creative Commons Attribution License, which permits unrestricted use, distribution, and reproduction in any medium, provided the original work is properly cited.