Research - (2021) Volume 11, Issue 3
Pollen in layers of seasonal snow in the South of Western Siberia
N. Kuryatnikova1*, N. Malygina2 and D. Pershin2,3Abstract
Snow cover affects and regulates many ecological patterns and processes. Pollen has been actively used to indicate ecosystem, cryosphere, and climatic processes and states. However, the relationships between pollen deposition and snow layering have not yet been sufficiently studied. In this work, we focus on assessing the interrelations between the taxa composition of pollen in different layers of the seasonal snow cover in the western Siberian forest-steppe ecoregion. The snowpack had a composite profile consisting of crusts and layers of faceted crystals and deep hoar between them. The lower deep layer of the hoar represented the maximum diversity of pollen grains (Pinus sp., Artemisia sp., Chenopodiaceae). Pollen transfer likely occurred when the surrounding territories (potential sources of secondary pollen input) were still open from the snow. In addition, we revealed that ice and melt-freeze crusts could prevent redistribution of pollen grains in the snowpack during winter, thereby providing a high taxonomic diversity. The five recognized taxa (Betula sp., Pinus sp., Artemisia sp., Asteraceae, and Chenopodiaceae) were identified between two layers of crust.
Keywords
Seasonal snow, pollen, forest steppe, western siberia
Introduction
Snow cover regulates many ecological patterns and processes (Slatyer et al., 2021). Snow transmits and modifies interactions between microorganisms, plants, animals, nutrients, the atmosphere, and soil (Snow ecology, 2001). The duration and amount of snow cover affect tree growth (Wu et al., 2018), vegetation productivity during the early growing season (Wang et al., 2017), soil microbial activity (Gavazov et al., 2017), and species distribution (Niittynen & Luoto, 2018). Furthermore, many species use the space under the snow cover as habitats (subnivean habitats) (Soininen et al., 2015; Guiden, Orrock, 2020). However, climate change has significantly affected the duration of snow cover and other snow characteristics (Pulliainen et al., 2020). These changes determine many challenges for ecosystems, such as loss of biodiversity and changes in the length of the growing season (Huss et al., 2017; Niittynen et al., 2018).
Pollen is a valuable indicator of ecological, climatic, and cryospheric processes. Pollen emission (Pollen Index) was used to study the temporal dynamics of high-mountain Mediterranean grasslands (Algarra et al., 2019). Airborne pollen has long been applied to track air masses and climate change (Ghasemifard et al., 2020; Sarda Estève et al., 2018, Clot, 2003). Pollen has been successfully implemented to distinguish between seasonal and annual layers of firn and ice cores (Nakazawa et al., 2005; Festi et al., 2017). However, the interactions between pollen deposition and seasonal snow metamorphism have not been sufficiently investigated. As shown for seasonal snow cover in Japan, the peak pollen concentration peak was consistently observed at the snowpack's surface during the melting period (Nakazawa and Suzuki, 2008). In other words, the maximum pollen concentration was not redistributed to the lower layers due to percolation. However, the behavior of pollen grains in regions with other snow types (eg, primarily temperature gradient metamorphism) needs to be clarified.
The concentrations of light-absorbing particles, such as black carbon and dust, in the snow cover have received enormous attention in recent years (Shevchenko et al., 2010; Meinander et al., 2020; Zhang et al., 2018). The presence of such particles in the snow can darken the snow surface, affect the energy balance, and further accelerate snow and ice melting (Kang et al., 2020; Hadley & Kirchstetter, 2012). For example, the effect of black carbon and dust reduced the duration of snow cover duration by 3.1 ± 0.1 to 4.4 ± 0.2 days on the Tibetan Plateau, China (Zhang et al., 2018). In this regard, pollen as an important indicator can be used to trace the possible origin of contaminants (Hicks & Isaksson, 2006).
Understanding the interactions between pollen deposition and snow layering is essential when applying pollen as an indicator of ecological, climatic, and cryospheric processes. In this study, we explore pollen relationship between the composition of pollen in different layers of seasonal snow cover in the south of western Siberia.
Study area
Snow profiling and pollen sampling were carried out on the Ob plateau occupying the southernmost part of the western Siberian plain (Fig. 1). The study area belongs to the West Siberian Forest Steppe ecoregion (Olson et al., 2011). The long stripes of pine
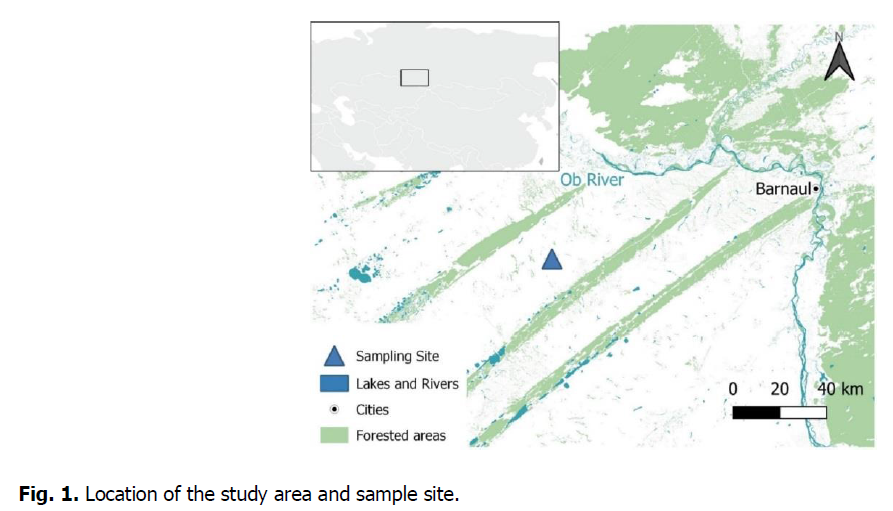
Fig 1: Location of the study area and sample site.
forests (Pinus sylvestris) are a distinctive feature of the Ob plateau. The forests are located within an extended ancient flow depression (Rudaya et al., 2020). However, the dominant type of land cover is arable land. Therefore, the sampling site was located within an extensive area of arable land representative of the surrounding area.
Snow profiling and samples
Snow profiling was conducted during the peak snow accumulation (March 2020) according to the International Classification for Seasonal Snow on the Ground (ICSSG) (Fierz et al., 2009). We characterized standard snowpack parameters: total snow height, bulk density, layering, grain size, grain shape, hand hardness, and snow temperature. Field data was analyzed and plotted using NiViz software (https://niviz.org/). We used data from the Rebrikha weather station (RIHMI–WDC database) to estimate snow accumulation conditions during winter of 2019-2020. Meteorological and pollen data were processed and plotted using R (https://www.r-project.org/).
Snow samples for pollen analysis were taken layer by layer using a snow tube sampler. The sampling sites were located in open areas at a considerable distance from trees (to exclude secondary contamination). The layers of samples were placed in plastic bags, labeled, and transported to the laboratory.
Identification of pollen
During the preparation of the primary sample, the samples were melted in plastic bags at room temperature, then we poured water into containers of suitable volume and added 40% formalin to reduce the microflora. Next, according to hydrobiological methods (Manual..., 1992), the samples were placed in a dark, cool place for 7-10 days to settle the sediment, after which it was separated by decantation.
Pollen samples were mounted and counted at a magnification of x 400 with the aid of a Laboval 4 light microscope (Carl Zeiss, Germany). We used a 0.2 ml Nageotte Counting Chamber to estimate the concentration of particles. The resulting samples were installed in aluminum sample holders, dried, and sputered by gold-palladium mixture in a SC7620 Mini Sputter Coater (Quorum Technologies Ltd, UK) for further electron microcopy. The use of a scanning electron microscope (SEM S-3400N Hitachi ScienceSystems Ltd, Japan (magnification up to 300000 resolution up to 3.0 nm) made it possible to detect pollen grains visually. For each slide, regular latitudinal sweeps were read. Pollen grains were identified according to atlases (Kupriyanova & Aleshina, 1972, 1978; Dzyuba, 2005; Karpovich et al., 2015) and international databases (https://www.paldat.org/search/A, https://pollenatlas.net/homepage).
Results and Discussion
We identified pollen grains from trees (Betula sp., Pinus sp.) and grasses (Artemisia sp., Asteraceae, Chenopodiaceae) in snow samples (Fig. 2). Pollen grains had a presumably advective origin, that is, they were transported from surrounding regions free of snow. The predicted origin was very likely since we sampled snow during peak accumulation (March 2020). The study area was fully covered with snow in that period, limiting the secondary transfer of pollen from the ground surface. Different transfer ranges characterize identified pollen grains (Sofiev et al., 2013). Pollen from trees belonging to wind-pollinated (anemophilous) plants is transported over considerable distances compared to insect-pollinated (entomophilous) taxa (most often grasses). For example, due to the morphology of the pollen grains (presence of air sacs), pine pollen may be transported up to several thousand kilometers, while birch pollen travels not more than 1000 km (Giner et al., 2001).

Fig 2: Identified pollen grains: a) Betula sp.; b) Pinus sp.; c) Artemisia sp.; d) Chenopodiaceae; e) Asteraceae.
Likewise, grass pollen is distributed within the areal, but under the influence of convective air flows, it may rise to the upper borders of the atmosphere's boundary layer and be transported over considerable distances. In the pollen spectrum obtained, 64% of pollen grains belonged to trees, with pine dominance (Fig. 3). Birch pollen was twice less than the pine–42%. Grasses were represented in approximately equal amounts from 14 to 7%.
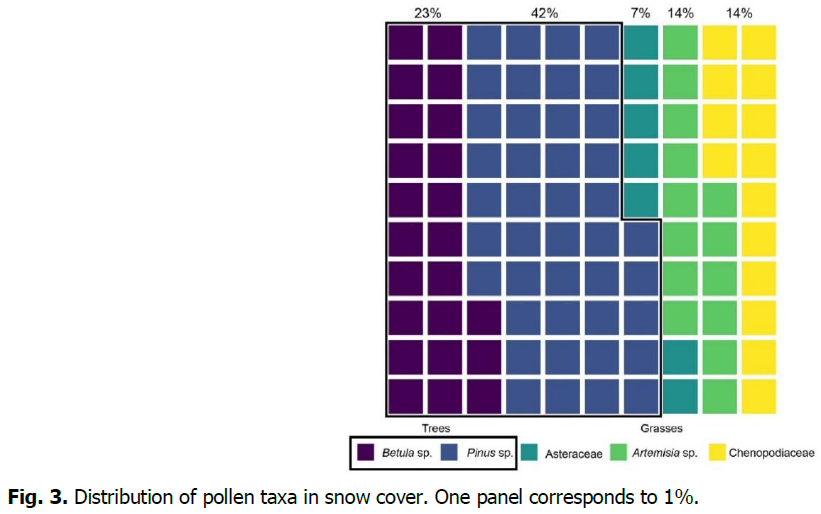
Fig 3: Distribution of pollen taxa in snow cover. One panel corresponds to 1%.
Snowpack stratigraphy was typical for moderately cold grassland regions, despite snow depth being significantly above average for this territory (Fig. 4). The profile consisted of several crusts and wind slabs separating faceted crystals and deep hoar (Fig. 5), which are layers standard for temperature gradient metamorphism (Sturm et al., 1995). However, many crusts and ice layers reflected warm periods during winter and the active influence of wind transport.
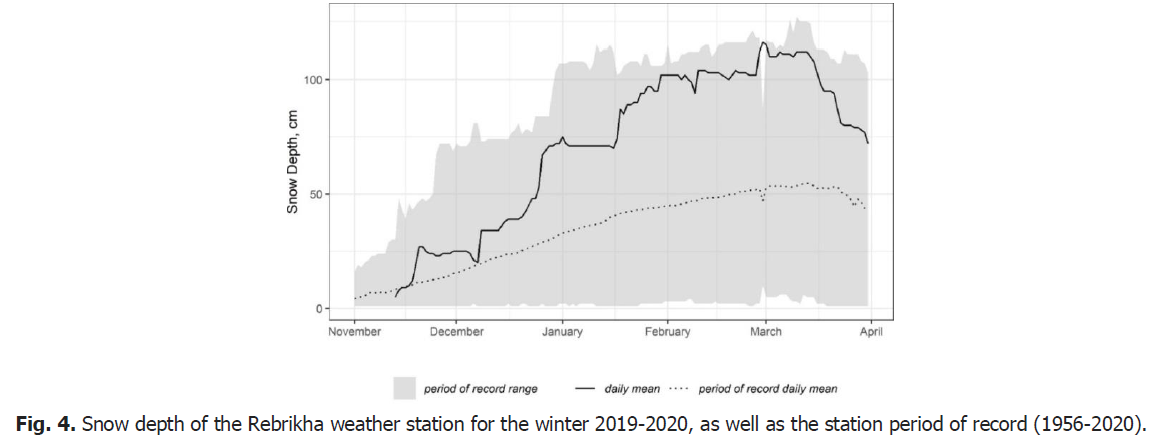
Fig 4: Snow depth of the Rebrikha weather station for the winter 2019-2020, as well as the station period of record (1956-2020).
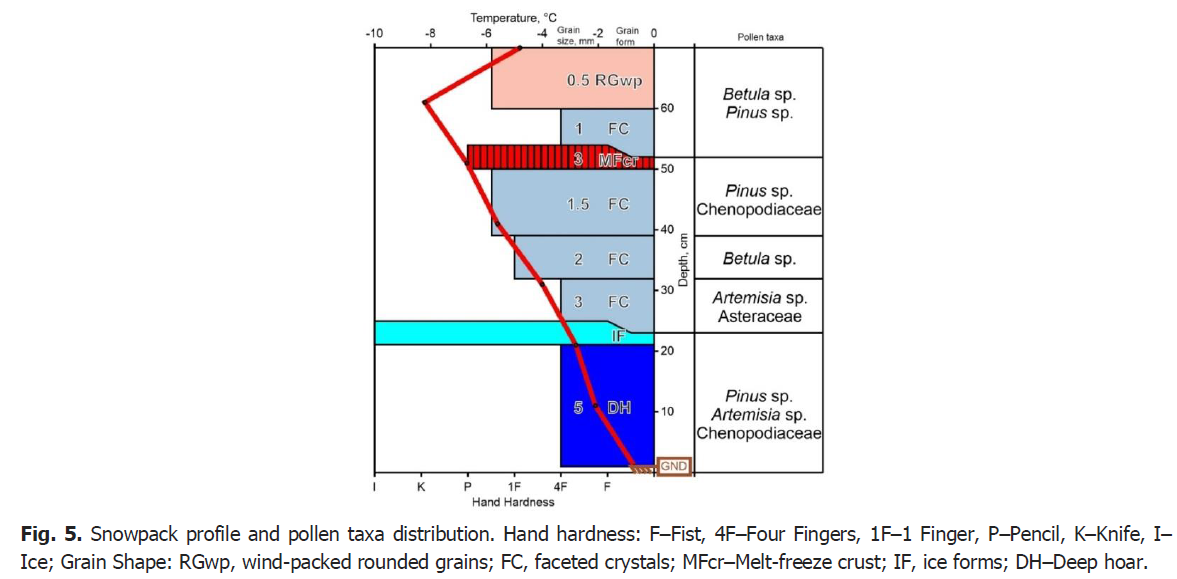
Fig 5: Snowpack profile and pollen taxa distribution. Hand hardness: F–Fist, 4F–Four Fingers, 1F–1 Finger, P–Pencil, K–Knife, I–Ice; Grain Shape: RGwp, wind-packed rounded grains; FC, faceted crystals; MFcr–Melt-freeze crust; IF, ice forms; DH–Deep hoar.
The highest taxonomic diversity (Pinus sp., Artemisia sp., Chenopodiaceae) in one layer was detected in the deep hoar (0–20 cm). This layer was formed at the beginning of the winter season when the surrounding southern regions were still free of snow cover. Secondarily, pollen grains could be transferred from these territories with air masses. Artemisia sp. and Chenopodiaceae identified in this layer are specific taxa of the steppes located in the southwest of the study area's southwest. The deep layer of hoar was overlain by an ice crust, which preserved pollen grains from wind redistribution.
In the faceted crystal layers located between the ice and melt-freeze crusts, all five recognized taxa were identified. Crusts appear to prevent the redistribution of pollen grains, that is, they contribute to pollen in the snowpack during winter. In the upper layer of wind that predominantly formed predominantly at the end of the winter period (end of February-March), the pollen of the trees was concentrated exclusively. It is more volatile compared to grass pollen and can remain in the atmosphere for a longer time.
Conclusion
The snowpack profile reflected the processes typical of cold steppe regions, the temperature gradient between the ground and the snow surface and the effect of wind transport. The influence of these processes formed a composite profile of crusts and layers of faceted crystals with deep hoar between them. In the snow pollen spectrum obtained, 64% of pollen grains belonged to trees, with pine dominance–42%. Grasses were represented in approximately equal amounts from 14 to 7%. The maximum diversity of pollen grains was represented in the lower layer of deep hoar, formed at the beginning of the winter season. This process probably occurred when the surrounding territories (potential sources of secondary pollen input) were still open from the snow. Furthermore, it was revealed that ice and melt-freeze crusts could prevent redistribution of pollen grains in the snow cover during the winter, thereby providing high taxonomic diversity: All five taxa were identified - trees (Betula sp., Pinus sp.) and grasses (Artemisia sp., Asteraceae, Chenopodiaceae).
Acknowledgements
Snow profiling was funded by RFBR, project number 19-35-60006. Pollen sampling and primary sample preparation were supported by RFBR, project number 19-35-90078. Meteorological analysis was funded by the Russian Science Foundation under grant number 21-17-00135. Microscopy analysis was carried out in "Shared research facilities of microscopy and X-ray spectroscopy of the Institute for Water and Environmental Problems, Siberian Branch of the Russian Academy of Sciences (Barnaul, Russia)."
References
Abakumov, V.A., Talskikh, V.N., Popchenko, V.I., Bulgakov, G.P., Svirskaya, N.L., Kryneva, S.V., Popchenko, I.I., Semin, V.A., Khromov, V.M., Raspopov, I.M., Margolina, G.L., Voronova, L.D., Pushkar, I.N. (1992). Manual for hydrobiological monitoring of freshwater ecosystems. Sankt-Peterburg, Gidrometeoizdat.
Algarra, J.A., Cariñanos, P., Herrero, J., Delgado-Capel, M., Ramos-Lorente, M.M., DÃaz de la Guardia, C. (2019). Tracking Montane Mediterranean grasslands: Analysis of the effects of snow with other related hydro-meteorological variables and land-use change on pollen emissions. Science of the Total Environment, 649:889â??901.
Clot, B. (2003). Trends in airborne pollen: An overview of 21 years of data in Neuchâtel (Switzerland). Aerobiologia, 19:227â??234. Deza-Araujo, M., Morales-Molino, C., Tinner, W., Henne, P.D., Heitz, C., Pezzatti, G.B., Conedera, M. (2020). A critical assessment of human-impact indices based on anthropogenic pollen indicators. Quaternary Science Reviews, p:236.
Domine, F. (2011). Physical properties of snow. Encyclopedia of Earth Sciences Series, pp:859â??863.
Dzjuba, O.F. (2005). Atlas of pollen grains (non-acetolized and acetolized), most common in the air basin of Eastern Europe. Moscow, p:70.
Estève, R.S., Baisnée, D., Guinot, B., Petit, J.E., Sodeau, J., O’Connor, D., Gros, V. (2018). Temporal variability and geographical origins of airborne pollen grains concentrations from 2015 to 2018 at saclay, France. Remote Sensing, 10:1932.
Festi, D., Carturan, L., Kofler, W., Fontana, G.D., De Blasi, F., Cazorzi, F., Oeggl, K. (2017). Linking pollen deposition and snow accumulation on the Alto dell’Ortles glacier (South Tyrol, Italy) for sub-seasonal dating of a firn temperate core. Cryosphere, 11:937â??948.
Fierz, C., Armstrong, R.L., Durand, Y., Etchevers, P., Greene, E., McClung, D.M., Nishimura, K., Satyawali, P.K. and Sokratov, S.A. (2009). The international classification for seasonal snow on the ground. ihp-vii technical documents in hydrology N°83, IACS Contribution N°1, UNESCO-IHP, Paris.
Frenz, D.A., Murray, L.W. (1997). An atmospheric pollen survey conducted during the winter in Minneapolis, Minnesota, USA. Grana, 36:245â??248.
Ghasemifard, H., Ghada, W., Estrella, N., Lüpke, M., Oteros, J., Traidl-Hoffmann, C., Menzel, A. (2020). High post-season Alnus pollen loads successfully identified as long-range transport of an alpine species. Atmospheric Environment, 231:117453.
Guiden, P.W., Orrock, J.L. (2020). Seasonal shifts in activity timing reduce heat loss of small mammals during winter. Animal Behaviour, 164:181â??192.
Hadley, O.L., Kirchstetter, T.W. (2012). Black-carbon reduction of snow albedo. Nature Climate Change, 2:437-440.
Hicks, S., Isaksson, E. (2006). Assessing source areas of pollutants from studies of fly ash, charcoal, and pollen from Svalbard snow and ice. Journal of Geophysical Research:Atmospheres.
Huss, M., Bookhagen, B., Huggel, C., Jacobsen, D., Bradley, R.S., Clague, J.J., Winder, M. (2017). Toward mountains without permanent snow and ice. Earth’s Future, 5:418â??435.
Kang, S., Zhang, Y., Qian, Y., Wang, H. (2020). A review of black carbon in snow and ice and its impact on the cryosphere. Earth- Science Reviews, 210:103346.
Karpovich, I.V., Drebezgina, E.S., Elovikova, E.N., Legotkina, G.I., Zubova, E.N., Kuzjaev, R.Z., Hismatullin, R.G. (2015). Pollen atlas. Ekaterinburg: Ural worker, Ñ?:318.
Kupriyanova, L.A., Aleshina, L.A. (1972). Pollen and plant spores of the flora of the USSR. Leningrad: Nauka, p:171.
Kupriyanova, L.A., Aleshina, L.A. (1978). Pollen and spores of dicotyledonous plants of the flora of the European part of the USSR.V.1. Leningrad: Nauka, Ñ?:174.
Meinander, O., Kontu, A., Kouznetsov, R., Sofiev, M. (2020). Snow samples combined with long-range transport modeling to reveal the origin and temporal variability of black carbon in seasonal snow in Sodankylä (67°N). Frontiers in Earth Science.
Nakazawa, F., Suzuki, K. (2007). The alteration in the pollen concentration peak in a melting snow cover. Bullettin of Glaciological Research, 25:1-7.
Nakazawa, F., Fujita, K., Takeuchi, N., Fujiki, T., Uetake, J., Aizen, V., Nakawo, M. (2005). Dating of seasonal snow/firn accumulation layers using pollen analysis. Journal of Glaciology, 51:483â??490.
Niittynen, P., Luoto, M. (2018). The importance of snow in species distribution models of arctic vegetation. Ecography, 41:1024â?? 1037.
Niittynen, P., Heikkinen, R.K., Luoto, M. (2018). Snow cover is a neglected driver of Arctic biodiversity loss. Nature Climate Change, 8:997â??1001.
Olson, D.M., Dinerstein, E., Wikramanayake, E.D., Burgess, N.D., Powell, G.V.N., Underwood, E.C., D'Amico, J.A., Itoua, I., Strand, H.E., Morrison, J.C., Loucks, C.J., Allnutt, T.F., Ricketts, T.H., Kura, Y., Lamoreux, J.F., Wettengel, W.W., Hedao, P., Kassem, K.R. (2001). Terrestrial ecoregions of the world: a new map of life on Earth. Bioscience, 51:933-938.
Pulliainen, J., Luojus, K., Derksen, C., Mudryk, L., Lemmetyinen, J., Salminen, M., Norberg, J. (2020). Patterns and trends of Northern Hemisphere snow mass from 1980 to 2018. Nature, 581:294â??298.
Rudaya, N., Krivonogov, S., SÅ?owiÅ?ski, M., Cao, X., Zhilich, S. (2020). Postglacial history of the Steppe Altai: Climate, fire and plant diversity. Quaternary Science Reviews.
Shevchenko, V.P., Korobov, V.B., Lisitzin, A.P., Aleshinskaya, A.S., Bogdanova, O.Y., Goryunova, N.V., Sapozhnikov, F.V. (2010). First data on the composition of atmospheric dust responsible for yellow snow in Northern European Russia in March 2008. Doklady Earth Sciences 431:497â??501.
Slatyer, R.A., Umbers, K.D.L., Arnold, P.A. (2021). Ecological responses to variation in seasonal snow cover. Conservation Biology. Sofiev, M., Belmonte, J., Gehrig, R., Izquierdo, R., Smith, M., Dahl, A., Siljamo, P. (2013). Airborne pollen transport. Allergenic Pollen, pp:127-159.
Soininen, E.M., Jensvoll, I., Killengreen, S.T., Ims, R.A. (2015). Under the snow: a new camera trap opens the white box of subnivean ecology. Remote Sensing in Ecology and Conservation, 1:29â??38.
Sturm, M., Holmgren, J., Liston, G.E. (1995). A seasonal snow cover classification system for local to global applications. Journal of Climate, 8:1261-1283.
Wang, X., Wang, T., Guo, H., Liu, D., Zhao, Y., Zhang, T., Piao, S. (2018). Disentangling the mechanisms behind winter snow impact on vegetation activity in northern ecosystems. Global Change Biology, 24:1651â??1662.
Wu, X., Li, X., Liu, H., Ciais, P., Li, Y., Xu, C., Zhang, C. (2019). Uneven winter snow influence on tree growth across temperate China. Global Change Biology, 25:144â??154.
Zhang, Y., Kang, S., Sprenger, M., Cong, Z., Gao, T., Li, C., Sillanpää, M. (2018). Black carbon and mineral dust in snow cover on the Tibetan Plateau. Cryosphere, 12:413â??431.
Author Info
N. Kuryatnikova1*, N. Malygina2 and D. Pershin2,32Institute for Water and Environmental Problems SB RAS, 1 Molodezhnaya St., Barnaul, 656038, Russia
3Lomonosov Moscow State University, 1 Leninskie Gory, Moscow, 119991, Russia
Citation: Kuryatnikova, N., Malygina, N., Pershin, D. (2021). Pollen in layers of seasonal snow in the South of Western Siberia. Ukrainian Journal of Ecology, 11 (3), 401-405.
Received: 12-May-2021 Accepted: 06-Jul-2021 Published: 24-Sep-2021, DOI: 10.15421/2021_0189
Copyright: This is an open access article distributed under the terms of the Creative Commons Attribution License, which permits unrestricted use, distribution, and reproduction in any medium, provided the original work is properly cited.