Research - (2022) Volume 12, Issue 4
Role of silicon foliar application in promoting salt stress tolerance and enhanced antioxidant capacity of Hordeum vulgare L. (cv. saida)
A. Alayat1,2*, Z. Boumedris1,2, S. Benosmane2,3, A. Atailia2,4, O. Moumeni2 and N. Hacini4Abstract
Barley production under salinity stress is a critical challenge facing many countries, particularly those in arid and semi-arid regions. Various methods were used for inducing salinity tolerance including application of stress tolerance-inducing substances. Silicon (Si) supplementation is considered one of the promising methods to improve plant resilience under salt stress. The aim of this study was to determine the imperative roles of silicon foliar application methods, in improving growth, malondialdehyde (MDA) superoxide (O2·-) and hydrogen peroxide (H2O2) contents and activating antioxidant system of salt affected barley plants. For this purpose, we investigated the effects of salinity (NaCl; 100 mM) stress on barley seedlings (Hordeum vulgare cv. saida) during silicon (Si; 1 mM and 2 mM) applications. The results showed that salinity had deleterious effects on plant growth. Salt-stressed plant shoots exhibited a greater activity of ascorbate peroxidase (APX), peroxidase (POD), but a lower activity of catalase (CAT) when compared to the control. Conversely, exogenous application of Si by spraying leaves led to improvements vegetative growth and caused significant increases in antioxidant defense systems of barley seedlings. Furthermore, lipid peroxidation, levels of superoxide, and hydrogen peroxide were decreased with silicon treatments. This results provides evidence of the positive influence of Si on alleviating the toxicity of NaCl in barley plants and can be further extended for field trials in salinity affected areas.
Keywords
Silicon, Salt stress, Hordeum vulgare, Lipid peroxidation, Reactive oxygen species, Antioxidants.
Introduction
Salinity stress is one of the most important environmental variables restricting the productivity of agricultural crops (Ahmad et al., 2019). Currently, more than 20% of the world’s agricultural irrigated land is affected by excess salt concentrations, which represents one third of food producing land (Gregory et al., 2018, Shrivastava and Kumar, 2015). The salt-affected areas are increasing at a rate of 10% annually for various reasons, including low precipitation, the weathering of native rocks, high surface evaporation, poor cultural practices, and irrigation using saline water (Shrivastava and Kumar, 2015). The drastic impacts of salinity stress on plant development are attributable to a hyperosmotic and ionic imbalance with excessive-production of reactive oxygen species (ROS) that severely hamper several physiological and biochemical pathways and molecular changes including chlorophyll depletion, lipid peroxidation, nucleic acid mutilation, and reduction in cell membrane fluidity and selectivity (Ren et al., 2020, Foyer, 2018, Parida and Das, 2005). The high concentration of reactive oxygen species produces several enzymatic antioxidants (e.g., catalase, peroxidase, and ascorbate peroxidase) to deal with the increase of ROS and protect the plant tissues from oxidative damage under various stresses (Al Mahmud et al., 2019, Liu et al., 2019).
Thus, plant nutrition has an important role to maintain healthy growth as well as to enhance the stress tolerance (Zargar et al., 2019, Bradacova et al., 2016, Vanderschuren et al., 2013). Silicon (Si) is one such nutritive element which is gaining increasing attention due to its observed properties enhancing plant tolerance against biotic as well as abiotic stresses (Ma, 2004). Moreover, Si can be regarded as multitalented element and could ameliorate soil conditions and nutrient contents (e.g., N, P, and K) in plants, making it a high-quality fertilizer for promoting ecologically sound agricultural practices (Zargar et al., 2019, Wu et al., 2015). Silicon can be regarded as one of the most important elements in agricultural production, particularly in justification of salinity stress effects, causes detrimental impacts for most plants grown in various global regions.
Barley (Hordeum vulgare L.) is the most salinity-tolerant species among the cereals, but it reacts negatively at higher concentrations (Munns and Tester, 2008). The foliar application of micronutrients including silicon seems to be the one of the methods of reducing the negative impact of salt stress on plants. This method of application is cheaper and more convenient to use than soil fertilization. The justification for feeding plants by application of liquid fertilizer containing silicon compounds directly to the leaves also exists because this element is not taken easily by the roots, e.g., in drought or saline conditions (Grašič et al., 2019, De Oliveira et al., 2019, Artyszak, 2018, Guével et al., 2007, Ma et al., 2004). Numerous studies conducted on many species of crops have proven the effectiveness of this method of application in creating plant resistance to environmental stresses such as high temperature, drought, salinity, and excess water (Stadnik et al., 2022; Niu et al., 2021, Abdelaal et al., 2020, Farouk et al., 2020, Babaeian et al., 2011, Sajedi et al., 2011).
Therefore, the present study aimed to assess the beneficial effects of silicon in barley seedlings (cv. saida) under control conditions, salt stress or their combination. Thus, the purpose to determine the effects of foliar application of silicon on plant growth, lipid peroxidation, up-regulation of antioxidant enzymes, and reactive oxygen species contents under salinity stress condition.
Materials and Methods
Plant material and growing conditions
Seeds of barley (Hordeum vulgare cv. saida) were kindly provided by the Algerian interprofessional office for cereals of Tebessa, Algeria. The seeds were surface sterilized in 5% Na(OCl)2 for 10 min, rinsed thoroughly with distilled water. The seeds rinsed thoroughly with distilled water, and germinated on moist filter paper for 3 days in an incubator at 25°C. After germination, the seeds were sown in 1.5 kg of soil with a grain size of clay sand and a slightly acidic pH (pH=6.5). The experiments were carried out in a greenhouse at a temperature of 22 ± 2°C, and a photoperiod of 16:8 h light: darkness. The experiment was conducted in a randomized block design with six replicates. The positions of the pots in the experiment were randomly changed every week. The composition of the basic nutrient solution was (mg.L-1): (NH4)2SO4 48.2, MgSO4 65.9, K2SO4 15.9, KNO3 18.5, Ca (NO3)2 59.9, KH2PO4 24.8, Fe citrate 6.8, MnCl2.4H2O 0.9, ZnSO4.7H2O 0.11, CuSO4.5H2O 0.04, H3BO3 2.9, H2MoO4 0.01. The solution was continuously aerated with air pumps and renewed every four days. In the sprout stage of plant growth (the stage of the first pair of leaves), a nutrient solution including NaCl with a concentration of 100 mM was applied once to the soil in each pot. Foliar application of Si was given in two concentrations of 1 mM and 2 mM. Spraying was performed with a laboratory hand sprayer with flow control of a dosing volume of 1.2 mL ± 0.1 during one press (outlet diameter 0.6 mm). Foliar application was applied via a uniform spraying procedure. Plants were sprayed until they were dripping. Nutrient solution was applied at the same time to the control pots. Plants in pots without NaCl (0 mM without addition of NaCl and Si) were used as controls. The experiment was a 2 × 2 factorial arrangements with two levels NaCl in nutrient solution, 0 mM (control) and 100 mM, and two levels of Si in nutrient solution, 1 and 2 mM, as Na2SiO3.9H2O. At 30 days after treatment, plants of each treatment were harvested, and shoot height and root length (mean of three longest roots in a plant) were measured.
Lipid peroxidation
The level of lipid peroxidation in plant tissues was expressed as 2-thiobarbituric acid (TBA) reactive metabolites, mainly malondialdehyde (MDA), and was determined according to Hodges et al., (1999). Both leaf samples for estimation of lipid peroxidation (MDA) were harvested at 30 days after treatment. Fresh samples (leaves) of around 0.5 g were homogenized in 4.0 ml of 1% trichloroacetic acid (TCA) solution and centrifuged at 10,000 × g for 10 min. The supernatant was added to 1ml 0.5% (w/v) TBA made in 20% TCA. The mixture was heated in boiling water for 30 min, and the reaction was stopped by placing the tubes in an ice bath. The samples were centrifuged at 10,000 × g for 10 min, and the absorbance of the supernatant was recorded at 532 nm. Correction of non-specific turbidity was made by subtracting the absorbance value read at 600 nm. The level of lipid peroxidation was expressed as n.mol g−1 fresh weight, with a molar extinction coefficient of 0.155 mM cm−1.
Determination of reactive oxygen species (superoxide anion and hydrogen peroxide)
The Hydrogen peroxide (H2O2) contents were assayed according to the method of Velikova et al., (2000). Leaves and roots were homogenized in ice bath with 0.1% (w/v) TCA. The extract was centrifuged at 12,000 × g for 15 min, after which to 0.5 mL of the supernatant was added 0.5 mL of 10 mM potassium phosphate buffer (pH 7.0) and 1 mL of 1 M KI and the absorbance was read at 390 nm. The content of H2O2 was given on a standard curve.
The detection of superoxide anion (O2-) is based on its ability to reduce a Nitro Blue Tetrazolium (NBT) group (Kubis, 2008). Shoots (0.1 g) were cut into 1 mm fragments and immersed in 10 mM potassium phosphate buffer (pH 7.8), containing 0.05% (w/v) NBT and 10 mM NaN3, and incubated for 1 h at room temperature. After incubation, 2 ml of the reaction solution was heated to 85°C for 15 minutes and rapidly cooled. The optical density was measured at 580 nm and the O2- content was expressed as an increase in absorption per dry weight.
Assay of antioxidant enzymes
Peroxidase (POD) activity was measured according to the method of Putter (1974), with some modification. The reaction mixture (3 ml) consisted of 100 µl enzyme extract, 100 µl guaiacol (1.5%, v/v), 100 µl H2O2 (300 mM) and 2.7 ml 25 Mm potassium phosphate buffer with 2 Mm EDTA (pH 7.0). Any increase in the absorbance due to oxidation of guaiacol was measured spectrophotometrically at 470 nm (ε=26.6mM−1 cm−1).
Ascorbate peroxidase activity (APX) was assayed according to the method of (Nakano and Asada, 1987). The reaction mixture consisted of 100 µl enzyme extract, 100 µl ascorbate (7.5 mM), 100 µl H2O2 (300 mM) and 2.7 ml 25 mM potassium phosphate buffer with 2 mM EDTA (pH 7.0). The oxidation of ascorbate was determined by the change in absorbance at 290 nm (ε=2.8mM−1 cm−1).
Catalase (CAT) activity was determinated according to Cakmak and Horst (1991). The reaction mixture for catalase in a total volume of 3 ml contained 50 mM Na-phosphate buffer (pH 7.2) and 300 mM H2O2. The reaction was started by adding enzyme extract and the activity was determined by monitoring the initial rate of H2O2 disappearance at 240 nm (ε=39.4/mM/cm).
Statistical analysis
All values reported in this study are the mean of at least three replicates. For each parameter, data were subjected to a one-way Anova analysis. When the effect was significant (p=0.05), differences between means were evaluated for significance by using Tukey’s (HSD) test (MINITAB software version 16.0).
Results
Plant growth
Effect of exogenous Si on the plant growth under salinity stress is presented in Fig. 1. In comparison to the control, salinity stress considerably decreased barley shoot and root length. Shoot and root length decreased 60% as a result of salinity (p<0.001). Inhibition in plant growth improved by addition of Si to the medium; however, it was still lower than the control plants. Moreover, when NaCl was absent from the nutrient media, Si significantly increased plant height (at 1 et 2 mM Si) relative to the control.
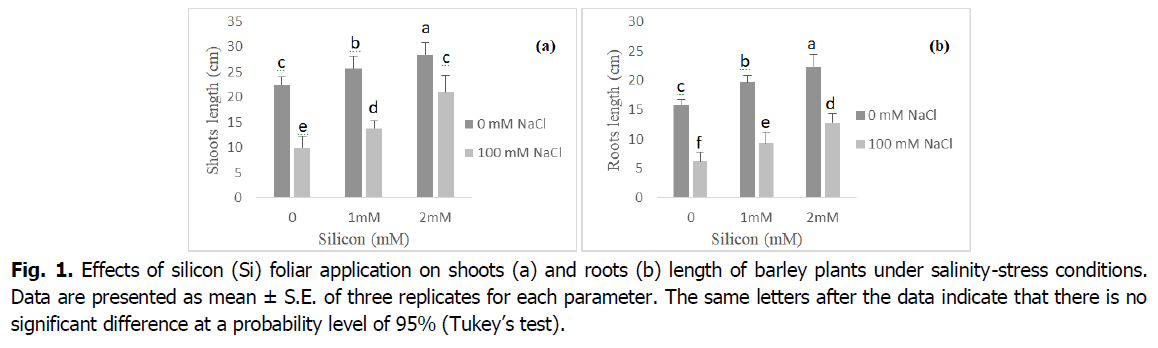
Fig 1. Effects of silicon (Si) foliar application on shoots (a) and roots (b) length of barley plants under salinity-stress conditions. Data are presented as mean ± S.E. of three replicates for each parameter. The same letters after the data indicate that there is no significant difference at a probability level of 95% (Tukey’s test).
Lipid peroxidation (MDA), superoxide anion and Hydrogen peroxide contents
The data for malondialdehyde (MDA) concentration are presented in Fig. 2. In shoots lipid peroxidation increased markedly under salinity (4.41 nmol/g Fw). However, foliar application of silicon significantly reduced MDA concentration (3.35 and 2.68 nmol/g Fw) of salt-stressed plants compared to the salt treatments alone (p<0.001).
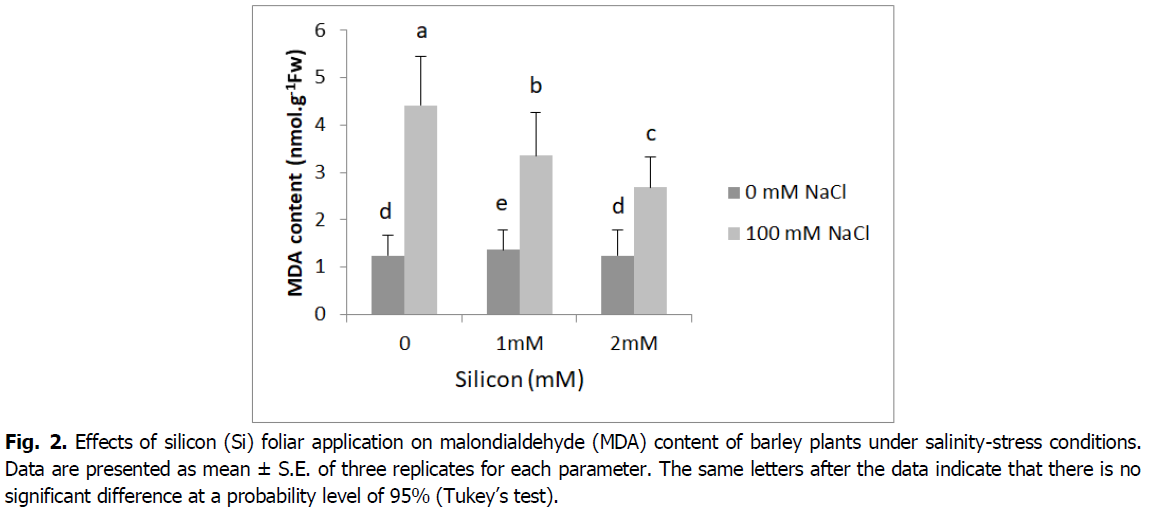
Fig 2. Effects of silicon (Si) foliar application on malondialdehyde (MDA) content of barley plants under salinity-stress conditions. Data are presented as mean ± S.E. of three replicates for each parameter. The same letters after the data indicate that there is no significant difference at a probability level of 95% (Tukey’s test).
The levels of superoxide anion significantly increased under salinity stress conditions (Fig. 3). Nevertheless, the silicon-treated salt-stressed barley plants showed significant decreases by 20% and 30% in superoxide anion production as compared to the salt stressed plants (p<0.001). Additionally, superoxide anion levels were decreased under foliar application of silicon compared to the untreated plants at both levels (1 and 2 mM) to relative control plants.
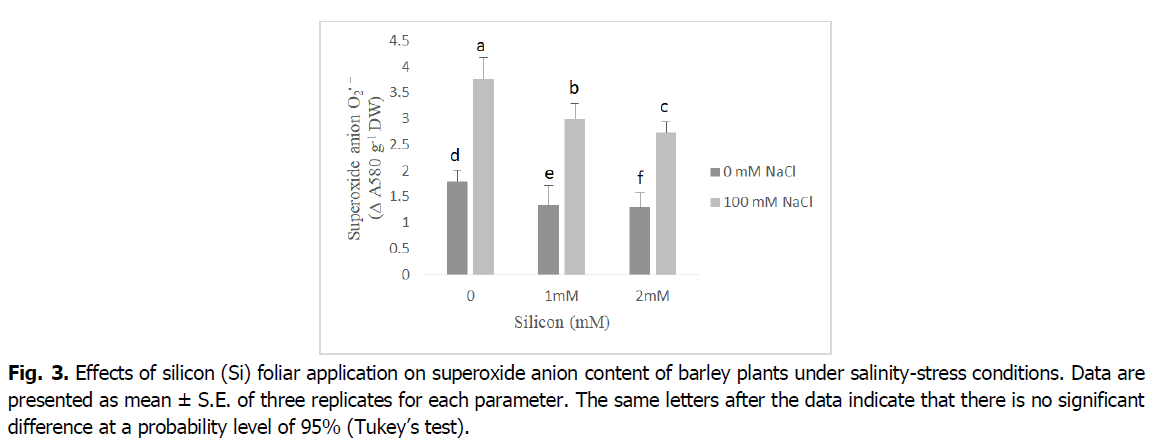
Fig 3. Effects of silicon (Si) foliar application on superoxide anion content of barley plants under salinity-stress conditions. Data are presented as mean ± S.E. of three replicates for each parameter. The same letters after the data indicate that there is no significant difference at a probability level of 95% (Tukey’s test).
The presented results in Fig. 4 show that salinity increased hydrogen peroxide (H2O2) production in barley shoots relative to untreated plants. The lowest concentration of H2O2 was noted in 2 mM Si plants treated, whereas plants that were grown under salinity accumulated the highest H2O2 concentration. In contrast, Si foliar application distinctively reduced NaCl-induced H2O2 accumulation in the shoots relative to salt-affected seedlings. Hence, this outcome was notable with Si foliar spraying at 2 mM that attenuated the impact of salinity, causing a 50% decline in H2O2 production relative to plants grown under salinity only (p<0.001).
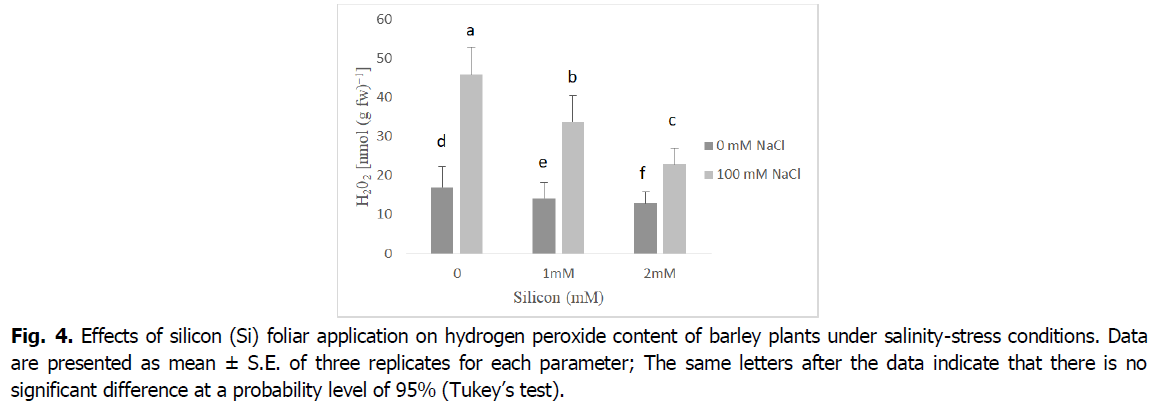
Fig 4. Effects of silicon (Si) foliar application on hydrogen peroxide content of barley plants under salinity-stress conditions. Data are presented as mean ± S.E. of three replicates for each parameter; The same letters after the data indicate that there is no significant difference at a probability level of 95% (Tukey’s test).
Antioxidant enzyme activities
The obtained results in Fig. 5 and 6 show that the antioxidant enzyme activities peroxidase (POD), and ascorbate peroxidase (APX) significantly increased in plants exposed to salinity stress relative to control seedlings (p<0.001). However, the foliar application of Si to barley seedlings under salt stress induced a significant decline in the activity of POD and APX enzymes when compared with the corresponding level of salinity. Barley seedlings treated with Si alone showed a decrease in the activity of POD and APX enzymes when compared with the control.
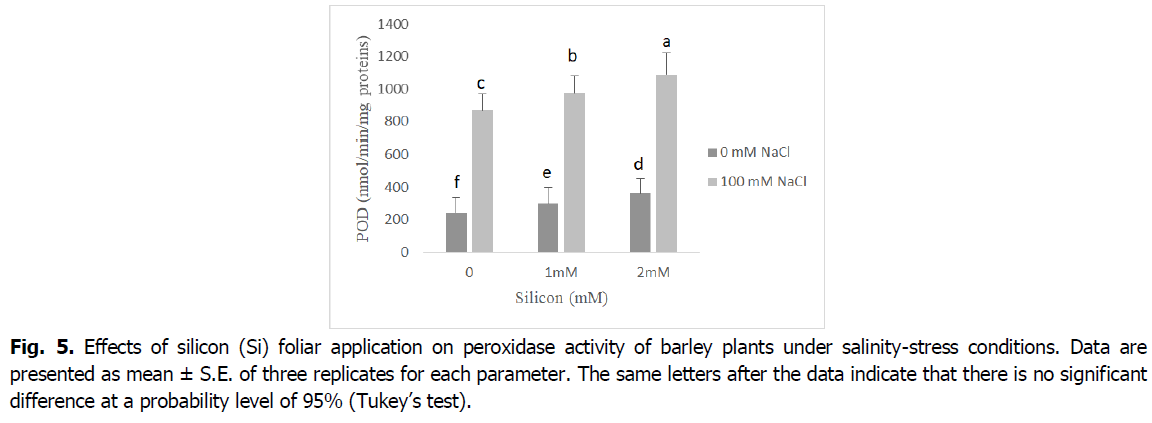
Fig 5. Effects of silicon (Si) foliar application on peroxidase activity of barley plants under salinity-stress conditions. Data are presented as mean ± S.E. of three replicates for each parameter. The same letters after the data indicate that there is no significant difference at a probability level of 95% (Tukey’s test).
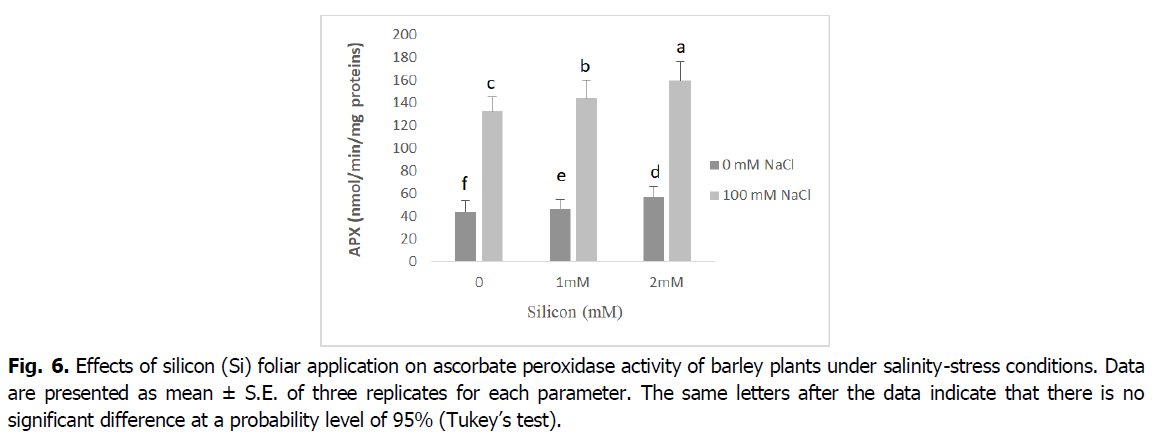
Fig 6. Effects of silicon (Si) foliar application on ascorbate peroxidase activity of barley plants under salinity-stress conditions. Data are presented as mean ± S.E. of three replicates for each parameter. The same letters after the data indicate that there is no significant difference at a probability level of 95% (Tukey’s test).
In contrast, CAT activity was significantly decreased in salt-stressed seedlings relative to control seedlings (p<0.001). However, the foliar application of Si to barley seedlings under salt stress ameliorated the inhibitory effect of NaCl by enhancing the activity of CAT enzyme compared with the corresponding level of salinity. Barley plants treated with either Si alone showed a significant increase in the activity of the CAT enzyme when compared with the control (Fig. 7).
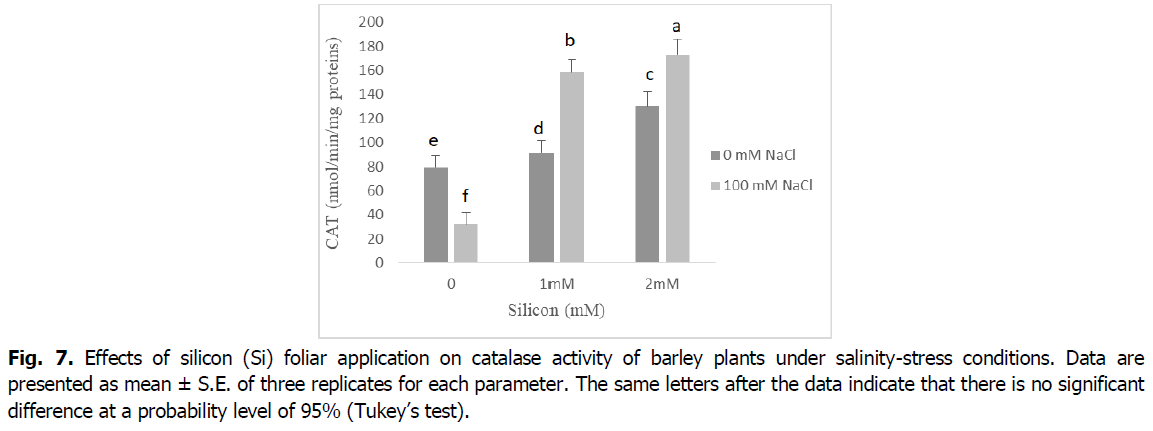
Fig 7. Effects of silicon (Si) foliarukrainian-journal-ecology-catalase-activity-12-4-28-g007 application on catalase activity of barley plants under salinity-stress conditions. Data are presented as mean ± S.E. of three replicates for each parameter. The same letters after the data indicate that there is no significant difference at a probability level of 95% (Tukey’s test).
Discussion
Salinity significantly reduced shoot and root growth of plants, however root tissues received more stress than shoots (Fig. 1). It might be due to the direct contact of root tips with stress. Thus, growth reduction due to salinity stress may be attributed to osmotic stress, ion imbalance and ion toxicity (Farouk and Al-Amri, 2019, Rios et al., 2017, Liu et al., 2015, Yazici et al., 2007). Our results have exhibited that growth characteristics were increased with Silicon supplementation. Indeed, according to Farouk and Omar (2002), the positive impacts of Si on plant growth may be due to the increased antioxidant capacity, and K+ absorption, which increase the number of chloroplasts per cell, and leaf area (Taiz and Zeiger, 2010). In several studies, foliar spray of silicon has been found to be effective to increase plant growth (Farouk et al., 2020, Al Murad et al., 2020, Farouk and Omar, 2020).
Lipid peroxidation, assayed as malondialdehyde (MDA) accumulation, significantly increased as a stress factor under salinity stress conditions (Fig. 2). This result could be due to the deleterious effect of salinity on barley plants, the damaging effect on membrane stability and selective permeability, consequently increasing electrolyte leakage (Abdelaal et al., 2020, El-Banna and Abdelaal, 2018). Our results indicate Si supplementation reduced considerably MDA production under salinity. However, the greatest decline in MDA content observed in plants under salinity that was subjected to Si foliar application (2 mM). This may indicate the potential of Si to counteract the harmful effects of salinity (Farouk et al., 2020). In maize, grapevine rootstock, rice and sweet pepper respectively Moussa (2006), Soylemezoglu et al. (2009), Kim et al. (2014) and Abdelaal et al. (2020) have reported that Si lowers the end-product of lipid peroxidation, i.e. malondialdehyde (MDA) content, therefore Si may help to maintain the integrity of cell membranes and lower their permeability (Coskun et al., 2016). Earlier reports indicated Si supplementation suppressed ROS damage by decreasing the levels of H2O2, superoxide anion and MDA, as well as by sustaining membrane integrity and activating antioxidant defense systems (Abdelaal et al., 2020, Farouk and Omar, 2020).
Reactive oxygen species, mainly hydrogen peroxide and superoxide anion, have considerably increased in salt-stressed barley plants (Fig. 3 and 4). The accumulation of superoxide and hydrogen peroxide under salinity stress is an important indicator of oxidative stress and plays a pivotal role in regulating the development and differentiation as well as stress signaling in plant organs (Alsaeedi et al., 2019, Shah et al., 2017, Khoshgoftarmanesh et al., 2014). The ROS overproduction is common in plants under salinity stress, which disturbs normal cellular processes (Siddiqui et al., 2020, Farouk and Al-Amri, 2019, Foyer, 2018, Parida and Das, 2005). Furthermore, in our results, we noted silicon application alleviates the harmful effects of salinity leading to decreased in levels of superoxide, and hydrogen peroxide. This may be due to the fact that silicon has an important role in regulating plasma membrane stability and increasing osmolyte accumulation which results in scavenging of reactive oxygen species, mainly hydrogen peroxide and superoxide (Shah et al., 2017). According to Al Murad et al. (2020), Siddiqui et al. (2020), Si treatment effectively attenuated the undesirable effects of oxidative stress on the cell membrane by acting as a ROS scavenger. Similar reports indicated Si supplementation suppressed ROS damage by decreasing the levels of H2O2, superoxide anion and MDA, as well as by sustaining membrane integrity and activating antioxidant defense systems (Farouk et al., 2020, Abdelaal et al., 2020, Farouk and Omar, 2020).
ROS scavengers such as catalase (CAT), ascorbate peroxidase (APX) and peroxidase (POD), are the most important osmoprotectors that prevent cellular oxidative damage during salt stress (Mansour and Ali, 2017). According to our results, roots and shoot APX and POD activities were enhanced by adding the NaCl to nutrient medium, and CAT activity decreased in this situation (Fig. 5 and 6). This may be a general adaptive defense response of plants to salinity at early stages. Several enzymes, such as catalase, ascorbate peroxidase and peroxidase, control intracellular H2O2 levels (Foyer, 2018). The increases of the enzymes APX and POD activities under salt stress are very important in dealing with the adverse effects of salinity by scavenging reactive oxygen species (Abdelaal et al., 2020). Similar results were obtained in many plants (El-Banna and Abdelaal, 2018, Wang et al., 2016).
The activity of the antioxidant enzymes CAT, POD and APX significantly boosted in the barley plants exposed to salinity stress following Si application. The exogenous application of silicon regulates antioxidant enzyme activities and maintains reactive oxygen species levels at nontoxic concentrations, protecting plants against oxidative damage under salinity conditions (Lin and Kao, 2000). The valuable influence of silicon in enhancing the activities of catalase, peroxidase, and ascorbate peroxidase enzymes as well as the elimination of superoxide and hydrogen peroxide could be due to the effect of silicon in controlling oxidative stress by alleviating ion toxicity and accumulation of nucleoproteins that contribute to plant resistance to stress factors (Abdelaal et al., 2020, Hernandez et al., 2010).
Conclusion
In conclusion, salinity stress has a negative impact on barley plants and this effect can be mitigated by foliar application of silicon. A positive effect of foliar spraying of Si was exhibited on improvements growth attributes and effectively alleviated the adverse effects of salt stress. Thus, silicon application enhanced all tested parameters such as membrane stability and activation of an antioxidant system to eliminate reactive oxygen species ROS. Indeed, the supplementation of Si to salinity stress initiates the development of resistance to stress in barley plants and may lead to an increase in yield potential in the future. Foliar application of Si can be an effective and environmentally friendly method of reducing the impact of soil salinity on crops.
References
Abdelaal, K., Mazrou, Y., Hafez, Y.M. (2020). Silicon foliar application mitigates salt stress in sweet pepper plants by enhancing water status, photosynthesis, antioxidant enzyme activity and fruit yield. Plants (Basel, Switzerland), 9:733.
Ahmad, R., Hussain, S., Anjum, M.A., Khalid, M.F., Saqib, M., Zakir, I., Hassan, A., Fahad, S., Ahmad, S. (2019). Oxidative stress and antioxidant defense mechanisms in plants under salt stress. In: Plant Abiotic Stress Tolerance (Springer), pp:191-205.
Al Mahmud, J., Bhuyan, M.H.M.B., Anee, T.I., Nahar, K., Fujita, M., Hasanuzzaman, M. (2019). Reactive oxygen species metabolism and antioxidant defense in plants under metal/metalloid stress. In: Hasanuzzaman, M., Hakeem, K.R., Nahar, K., Alharby, H.F., editors. Plant Abiotic Stress Tolerance. Springer International Publishing; Cham, Switzerland, pp:221-257.
Al Murad, M., Khan, A., Muneer, S. (2020). Silicon in horticultural crops: cross-talk, signaling, and tolerance mechanism under salinity stress. Plants, 9:460.
Alsaeedi, A., El-Ramady, H., Alshaal, T., El-Garawany, M., Elhawat, N., Al-Otaibi, A. (2019). Silica nanoparticles boost growth and productivity of cucumber under water deficit and salinity stresses by balancing nutrients uptake. Plant Physiology Biochemistry, 139:1-10.
Artyszak, A. (2018). Effect of silicon fertilization on crop yield quantity and quality-A literature review in Europe. Plants, 7:54.
Babaeian, M., Tavassoli, A., Ghanbari, A., Esmaeilian, Y., Fahimifard, M. (2011). Effects of foliar micronutrient application on osmotic adjustments, grain yield and yield components in sunflower (Alstar cultivar) under water stress at three stages. African Journal of Agriculture and Research, 6:1204-1208.
Bradacova, K., Weber, N.F., Morad-Talab, N., Asim, M., Imran, M., Weinmann, M., Neumann, G. (2016). Micronutrients (Zn/Mn), seaweed extracts, and plant growth-promoting bacteria as cold-stress protectants in maize. Chemical and Biological Technology and Agriculture, 3:19.
Cakmak, I., Horst, W.J. (1991). Effect of aluminium on lipid peroxidation, superoxide dismutase, catalase, and peroxidase activities in root tips of soybean (Glycine max). Physiology Plantarum, 83:463-468.
Coskun, D., Britto, D.T., Huynh, W.Q., Kronzucker, H.J. (2016). The role of silicon in higher plants under salinity and drought stress. Frontier Plants and Science, 7:1072.
De Oliveira, R.L.L., De Mello Prado, R., Felisberto, G., Cruz, F.J.R. (2019). Different sources of silicon by foliar spraying on the growth and gas exchange in sorghum. Journal of Soil Science and Plant Nutrition, 19:948-953.
El-Banna, M.F., Abdelaal, K.A.A. (2018). Response of strawberry plants grown in the hydroponic system to pretreatment with h2o2 before exposure to salinity stress. Journal of Plant Production, 9:989-1001.
Farouk, S., Khalid Elhindi, M., Majed Alotaibi, A. (2020). Silicon supplementation mitigates salinity stress on Ocimum basilicum L. via improving water balance, ion homeostasis, and antioxidant defense system. Ecotoxicology and Environmental Safety, 206:111396.
Farouk, S., Al-Amri, S.M. (2019). Exogenous zinc forms counteract NaCl-induced damage by regulating the antioxidant system, osmotic adjustment substances and ions in canola (Brassica napus L. cv Pactol) plants. Journal of Soil Science and Plant Nutrition, 19:887-899.
Farouk, S., Omar, M.M. (2020). Sweet basil growth, physiological and ultrastructural modification, and oxidative defense system under water deficit and silicon forms treatment. Journal of Plant Growth and Regulation, 39:1307-1331.
Foyer, C.H. (2018). Reactive oxygen species, oxidative signaling and the regulation of Photosynthesis. Environmental and Experimental Botany, 154:134-142.
Grašič, M., Dobravc, M., Golob, A., Vogel-Mikuš, K., Gaberščik, A. (2019). Water shortage reduces silicon uptake in barley leaves. Agricultural Water Management, 217:47-56.
Gregory, P.J., Ismail, S., Razaq, I.B., Wahbi, A. (2018). Soil salinity: current status and in depth analyses for sustainable use.
Guével, M.H., Menzies, J.G., Bélanger, R.R. (2007). Effect of root and foliar applications of soluble silicon on powdery mildew control and growth of wheat plants. Europe Journal of Plant Pathology, 119:429-436.
Hernandez, M., Fernandez-Garcia, N., Diaz-Vivancos, P., Olmos, E.A. (2010). Different role for hydrogen peroxide and the antioxidative system under short and long salt stress in Brassica oleracea roots. Journal of Experimental Botony, 61:521-535.
Hodges, D.M., DeLong, J.M., Forney, C.F., Prange, R.K. (1999). Improving the thiobarbituric acid-reactive-substances assay for estimating lipid peroxidation in plant tissues containing anthocyanin and other interfering compounds. Planta, 207:604-611.
Khoshgoftarmanesh, A.H., Khodarahmi, S., Haghighi, M. (2014). Effect of silicon nutrition on lipid peroxidation and antioxidant response of cucumber plants exposed to salinity stress. Architech and Agronomy of Soil Science, 60:639-653.
Kim, Y.H., Khan, A.L., Waqas, M., Shim, J.K., Kim, D.H., Lee, K.Y., Lee, I.J. (2014). Silicon application to rice root zone influenced the photo hormonal and antioxidant responses under salinity stress. Journal of Plant Growth Regulation, 33:137-149.
Kubis, J. (2008). Exogenous spermidine differentially alters activities of some scavenging system enzymes, H2O2 and superoxide radical levels in water stressed cucumber leaves. Journal of Plant Physiology, 165:397-466.
Lin, C.C., Kao, C.H. (2000). Effect of NaCl stress on H2O2 metabolism in rice leaves. Plant Growth Regulation, 30:151-155.
Liu, B., Soundararajan, P., Manivannan, A. (2019). Mechanisms of silicon-mediated amelioration of salt stress in plants. Plants (Basel), 8:27.
Liu, P., Yin, L., Wang, S., Zhang, M., Deng, X., Zhang, S., Tanaka, K. (2015). Enhanced root hydraulic conductance by aquaporin regulation accounts for silicon alleviated saltinduced osmotic stress in Sorghum bicolor L. Environmental and Experimental Botany.
Ma, J.F. (2004). Role of silicon in enhancing the resistance of plants to biotic and abiotic stresses. Soil Science and Plant Nutrition, 50:11-18.
Ma, J., Choi, H., Stafford, R.J., Miller, M.J. (2004). Silicone-specific imaging using an inversion-recovery-prepared fast three-point Dixon technique. Journal of Magnetic Resoning and Imaging, 19:298-302.
Mansour, M.M.F., Ali, E.F. (2017). Evaluation of proline functions in saline conditions. Phytochemistry, 140:52-68.
Munns, R., Tester, M. (2008). Mechanisms of salinity tolerance. Annual Review Plant Biology, 59:651-681.
Moussa, H.R. (2006). Influence of exogenous application of silicon on physiological response of salt-stressed maize (Zea mays L.). International Journal of Agriculture and Biology, 8:293-297.
Nakano, Y., Asada, K. (1987). Purification of ascorbate peroxidase in spinach chloroplasts; its inactivation in ascorbate depleted medium and reactivation by monodehydro ascorbate radical. Plant Cell Physiology, 28:131-140.
Niu, J., Liu, C., Huang, M., Liu, K., Yan, D. (2021). Effects of foliar fertilization: A review of current status and future perspectives. Journal of Soil Science and Plant Nutrition, 21:104-118.
Parida, A.K., Das, A.B. (2005). Salt tolerance and salinity effects on plants: a review. Ecotoxicology Environment Safety, 60:324-349.
Putter, J. (1974). Peroxidases. In: Bergmeyer, H.U. (Ed.), Methods of enzymaticanalysis: II. Academic Press. New York, pp:685-690.
Ren, J., Ye, J., Yin, L., Li, G., Deng, X., Wang, S. (2020). Exogenous melatonin improves salt tolerance by mitigating osmotic, ion, and oxidative stresses in maize seedlings. Agronomy, 10:663.
Rios, J.J., Martínez-Ballesta, M.C., Ruiz, J.M., Blasco, B., Carvajal, M. (2017). Silicon-mediated improvement in plant salinity tolerance: The role of aquaporins. Frontier Plant Science, 8:948.
Sajedi, N.A., Ardakani, M.R., Madani, H., Naderi, A., Miransari, M. (2011). The effects of selenium and other micronutrients on the antioxidant activities and yield of corn (Zea mays L.) under drought stress. Physiology and Molecular Biological Plants, 17:215-222.
Shah, S., Houborg, R., McCabe, M. (2017). Response of Chlorophyll, Carotenoid and SPAD-502 Measurement to Salinity and Nutrient Stress in Wheat (Triticum aestivum L.). Agronomy, 7:61.
Shrivastava, P., Kumar, R. (2015). Soil salinity: a serious environmental issue and plant growth promoting bacteria as one of the tools for its alleviation. Saudi Journal of Biological Sciences, 22:123-131.
Siddiqui, M.H., Alamri, S., Alsubaie, Q.D., Ali, H.M., Khan, M.N., Al-Ghamdi, A., Ibrahim, A.A., Alsadon, A. (2020). Exogenous nitric oxide alleviates sulfur deficiency-induced oxidative damage in tomato seedlings. Nitric Oxide Biological Chemistry, 94:95-107.
Soylemezoglu, G., Demir, K., Inal, A., Gunes, A. (2009). Effect of silicon on antioxidant and stomatal response of two grapevine (Vitis vinifera L.) rootstocks grown in boron toxic, saline and boron toxic-saline soil. Scientia Horticulture, 123:240-246.
Stadnik, B., Tobiasz-Salach, R., Mazurek, M. (2022). Physiological and epigenetic reaction of Barley (Hordeum vulgare L.) to the foliar application of silicon under soil salinity Conditions. International Journal of Molecular Sciences, 23:1149.
Taiz, L., Zeiger, E. (2010). Plant Physiology. Sinauer Associates Inc, Sunderlands, USA.
Vanderschuren, H., Boycheva, S., Li, K.T., Szydlowski, N., Gruissem, W., Fitzpatrick, T.B. (2013). Strategies for vitamin B6 biofortification of plants: a dual role as a micronutrient and a stress protectant. Frontier Plant Science, 4:143.
Velikova, V., Yordanov, I., Edreva, A. (2000). Oxidative stress and some antioxidant systems in acid rain-treated bean plants-protective role of exogenous polyamines. Plant Science, 151:59-66.
Wang, B., Zhang, M., Fu, R., Qian, X., Rong, P., Zhang, Y., Jiang, P., Wang, J., Lu, X., Wang, D. (2016). Epigenetic mechanisms of salt tolerance and heterosis in upland cotton (Gossypium hirsutum L.) revealed by methylation-sensitive amplified polymorphism analysis. Euphytical, 208:477-491.
Wu, J., Guo, J., Hu, Y., Gong, H. (2015). Distinct physiological responses of tomato and cucumber plants in silicon-mediated alleviation of cadmium stress. Frontier Plant Science, 6:453.
Yazici, I., Turkan, I., Sekmen, A.H., Demiral, T. (2007). Salinity tolerance of purslane (Portulaca oleracea L.) is achieved by enhanced antioxidative system, lower level of lipid peroxidation and proline accumulation. Environmental and Experimental Botany, 61:49-57.
Zargar, S.M., Mahajan, R., Bhat, J.A., Nazir, M., Deshmukh, R. (2019). Role of silicon in plant stress tolerance: opportunities to achieve a sustainable cropping system. Biotechnology, 9:73.
Author Info
A. Alayat1,2*, Z. Boumedris1,2, S. Benosmane2,3, A. Atailia2,4, O. Moumeni2 and N. Hacini42LTC Laboratory, Faculty of Life and Natural Sciences, Badji Mokhtar University, Annaba, Algeria
3Faculty of Natural and Life Sciences and Earth and Universe Sciences, University 8 Mai 1945 Guelma, Algeria
4LFEE Laboratory, Faculty of Life and Natural Sciences, Chadli Bendjedid University, El Tarf, Algeria
Citation: Alayat, A., Boumedris, Z., Benosmane, S., Atailia, A., Moumeni, O., Hacini, N. (2022). Role of silicon foliar application in promoting salt stress tolerance and enhanced antioxidant capacity of Hordeum vulgare L. (cv. saida). Ukrainian Journal of Ecology. 12:28-35.
Received: 18-Mar-2022, Manuscript No. UJE-22-57749; , Pre QC No. P-57749; Editor assigned: 21-Mar-2022, Pre QC No. P-57749; Reviewed: 02-Apr-2022, QC No. Q-57749; Revised: 08-Apr-2022, Manuscript No. R-57749; Published: 16-Apr-2022, DOI: 10.15421/2022_362
Copyright: This is an open access article distributed under the terms of the Creative Commons Attribution License, which permits unrestricted use, distribution, and reproduction in any medium, provided the original work is properly cited.