Research - (2021) Volume 0, Issue 0
Sclerotic productivity, mycelial compatibility, and pathogenicity of the isolates of the fungus Sclerotinia sclerotiorum (Lib.) de Bary
M. Kyryk1, M. Pikovskyi1, O. Kolesnichenko1, V. Borodai1, O. Markovska2*, V. Dudchenko2 and M. Solomiyciuk3Abstract
The study aimed to determine the variability of S. sclerotiorum populations from different soil and ecological conditions of Ukraine. Using phytopathological and mycological methods, 42 isolates of S. sclerotiorum, the causative agent of white mold, were obtained from infected soybean, sunflower, dahlia, winter rape and pea plants and stored on potato-glucose agar (PGA). To study sclerotic productivity, isolates were cultured on PGA in Petri dishes. To identify groups of mycelial compatibility, isolates were grown in pairs in all possible combinations. To study the pathogenicity of S. sclerotiorum isolates, leaves of winter rape were inoculated with 5 mm disks of mycelial culture from a five-day-old fungus, and surface areas of lesions on the leaf blade were measured. According to the results of the study, intensive production of sclerotia is exhibited by isolates of S. sclerotiorum from the population G. The smallest mass of sclerotia was produced by Bn28 (0.15 g), D21 (0.17 g), and D25 (0.19 g) isolates. Han10 and Han 40 (0.43 g), D26 (0.44 g), and Han 39 (0.46 g) isolates were the most productive. Mass sclerotia produced by all other investigated isolates of S. sclerotiorum ranged from 0.21 g to 0.37 g. Variability in mycelial compatibility of different combinations of S. sclerotiorum isolates was also revealed. Isolates obtained from soybean plants (population A) were characterized by the lowest level of mycelial compatibility-14%. In population C (sunflower), the proportion of isolate pairs characterized by mycelial compatibility was the largest-67%. All studied S. sclerotiorum isolates obtained from various host plants caused pathological processes on leaves of winter rape. Han42, Ps37, D21, and Han38 isolates had the lowest levels of pathogenicity. Han8, Han12, Han17, Han16, Gm6, Bn28, and Ps33 isolates were characterized by moderate pathogenicity. Strong pathogenicity was shown by Han18, D24, Gm2, Ha13, D22, D27, Han14, Ps35, Ps35, Han9, Han11, D23, D26, Han39, Gm5, Han20, and Bn32 isolates. Han40, Han10, Gm3, Gm7, Han19, Han41, D25, Bn31, Gm1, Ps34, Gm4, Bn29, and Bn30 isolates had a very high degree of pathogenicity. The study results revealed that the fraction of compatible pairs of isolates in different populations ranged from 14 to 67%.
Keywords
White mold, isolation, populations, sclerotia, mycelial compatibility, pathogenicity.
Introduction
The necrotrophic ascomycete Sclerotinia sclerotiorum (Lib.) de Bary is a cosmopolitan fungus that infects 408 plant species belonging to 275 different families, causing sclerotiniosis, also known as white mold (Boland & Hall, 1994). This disease leads to a shortage of oilseeds, legumes, vegetables (Derbyshire et al., 2016; Gulya et al., 2018; Barro et al., 2019; Mamadou et al., 2017; Kirik et al., 2001) and can cause loss of decorative properties or death of flowering plants (Pikovskyi et al., 2019).
S. sclerotiorum is capable of vegetative and sexual reproduction. The latter occurs via germination of sclerotia with subsequent formation of mycelium (Lane et al., 2018; Lane et al., 2019), while the former-via carpogenic germination of sclerotia leads to the formation of apothecia that produce ascospores. As the fungus is homotal, ascospores can support its entire life cycle. Both types of reproduction lead to the dominance of the clonal population structure. However, asexual reproduction is more common in nature (Winton et al., 2006). It is known that sexual reproduction (Foley et al., 2016; Willbur et al., 2017) in fungal populations can increase genetic diversity and adaptability of the pathogen (Hemmati et al., 2009; Attanayake et al., 2014). Aldrich-Wolf L. and Travers S. (Aldrich-Wolfe & Travers, 2015) studied the genetic variation of S. sclerotiorum in the north-central United States and found that reproduction among its isolates was primarily clonal. However, genetic recombination in isolates from rape and sunflower plants was reported.
The study and identification of mycelial compatibility groups, which are controlled by several loci in fungi, is a typical phenotypic, macroscopic analysis (Carbone et al., 2009). Konh et al. (1991) indicated that mycelial incompatibility characterizes the inability of different strains to merge and form one colony and causes cell death and reduced growth of two incompatible colonies. One of the criteria for detecting clonality is grouping according to mycelial compatibility. If isolates (strains) combine in culture and represent compatible mycelial groups, they can form anastomoses and generate a single fused colony without a reaction line. They also have a unique and complex DNA fingerprint (Carbone et al., 1999; Kohn et al., 1991).
Studies conducted in several countries enabled segregation of clonal isolates of S. sclerotiorum into groups of mycelial compatibility and led to determination of morphological polymorphisms and pathogenic diversity (Dalili et al., 2015; Lehner et al., 2016; Tok et al., 2016; Sharma et al., 2018; Liu et al., 2018; Rahman et al., 2020). The variability of S. sclerotiorum isolates from the phyllosphere of plants from different conditions in Ukraine has not been previously explored. Therefore, the study of pathogen variability in specific soil and climatic conditions is essential for assessing the status of populations of the causative agent of white mold in plants.
Materials and Methods
The study aimed to determine the variability of S. sclerotiorum populations from different soil and ecological conditions of Ukraine. Hence, the following goals were set: to characterize the natural populations of S. sclerotiorum according to their ability to produce sclerotia; to assess mycelial compatibility groups (MCGs); to study the pathogenic properties of fungal isolates obtained from various host plants and populations.
Laboratory research was conducted in "Mycology and Phytopathology" research laboratory of the National University of Life and Environmental Sciences of Ukraine. The objects of the study were isolates of S. sclerotiorum, obtained from the phyllosphere of infected soybean, rape, sunflower and dahlia plants grown under different soil and climatic conditions. First, sclerotia obtained from infected plants were superficially sterilized in 70% ethyl alcohol for 2 minutes, washed in sterile distilled water, dried between layers of sterile filter paper, and aseptically transferred into Petri dishes on potato-glucose agar (PGA). Then, they were incubated in a thermostat at 22-24°C for three days in the dark. From each isolate, tips of hyphal cultures of the fungus were seeded onto aqueous agar (AA), which was prepared using 1 liter of distilled water and 20 g of agar-agar. After that, the isolates were cultured on PGA and stored in a refrigerator at 4-5°C for further experiments (Rathi et al., 2018). In total, 42 isolates of S. sclerotiorum were obtained and divided into populations (Table 1).
Populations | Isolates | Crop | Isolation site |
---|---|---|---|
А | Gm1, Gm2, Gm3, Gm4, Gm5, Gm6, Gm7 | Soybean (Glycine max L.) | Kyiv region, Vasylkiv district |
B | Han8, Han9, Han10, Han11, Han12, Han13, Han14 | Sunflower (Helianthus annuus L.) |
Zhytomyr region, Popilnyanskyi district |
C | Han15, Han16, Han17, Han18, Han19, Han20 | Sunflower (Helianthus annuus L.) |
Kyiv region, Bila Tserkva district |
D | D21, D22, D23, D24, D25, D26, D27 | Dahlias (Dahlia Сav.) |
Kyiv, Holosiivskyi district |
E | Bn28, Bn29, Bn30, Bn31, Bn32 | Winter rape (Brassica napus L.) |
Kyiv region, Vasylkiv district |
F | Ps33, Ps34, Ps35, Ps36 Ps37 | Peas (Pisum sativum L.) |
Ivano-Frankivsk region, Horodenka district |
G | Han38, Han39, Han40, Han41, Han42 | Sunflower (Helianthus annuus L.) |
Kyiv region, Yahotyn district |
Table 1. Isolates of S. sclerotiorum were obtained from regions of conducted studies.
To study sclerotic productivity, isolates were cultured on PGA in Petri dishes with a diameter of 90 mm in triplicates at temperatures of 22-23°C. After 30 days, sclerotia were removed from Petri dishes, dried at room temperature for 48 hours, and determined their number and weight (Morrall et al., 2011).
To assess MCGs, agar disks with a diameter of 5 mm were curved out with a stopper drill from the edge of an actively growing colony of S. sclerotiorum from a four-day-old isolate culture. Subsequently, the discs were placed in pairs on PGA [Schafer and Kohn 2006]. All possible combinations of isolates were tested. Assessment of compatibility or incompatibility was performed 10 days after incubation of cultures at a temperature of 24°C. The isolates were classified as compatible when the reaction line was not observed in the zone of mycelial interaction (Figure 1a) and vice versa (Figure 1b) (Kohn et al., 1990).
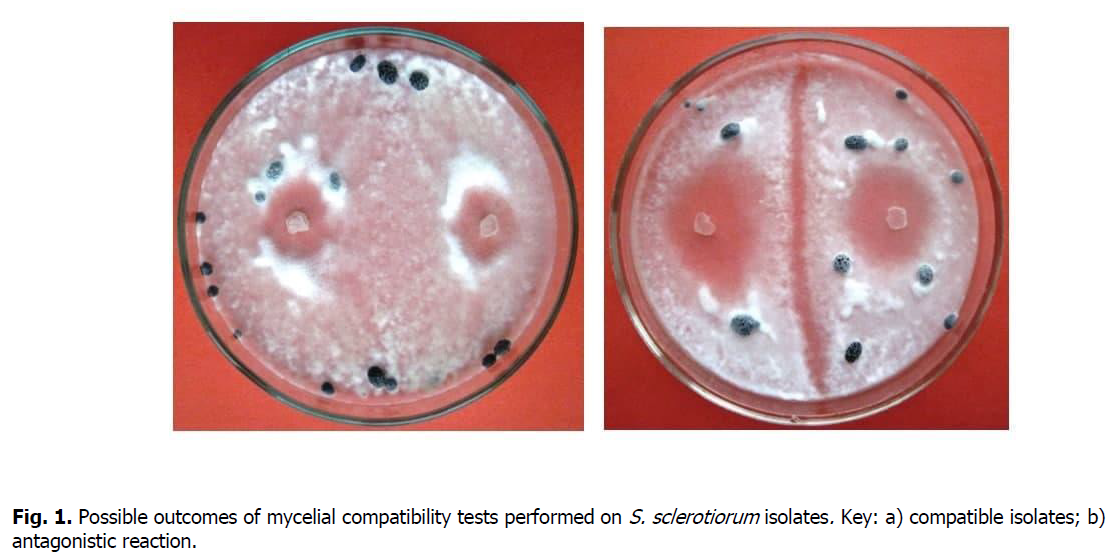
Figure 1: Possible outcomes of mycelial compatibility tests performed on S. sclerotiorum isolates. Key: a) compatible isolates; b) antagonistic reaction.
To study the pathogenicity of S. sclerotiorum isolates, winter rape leaves (Sokolivsky hybrid grown under controlled conditions) were used at the rosette stage. The leaves were separated from the parent plant and placed in sterile plastic germination dishes containing glass rods at the bottom, which were covered with sheets of filter paper that were soaked in sterile water beforehand. 5 mmdisks of the mycelial culture from five-day-old fungi grown on PGA were placed in the center of the leaves, on the central vein. The top of the germination dishes was covered with clear plastic. Inoculated leaves were kept at a temperature of 23 ± 2°C, with a photoperiod of 12 hours (Zanatta et al., 2019). The experiment was repeated four times. Pathogenicity was recorded on the 5th day of inoculation. Characteristic symptoms of the disease were wet spots saturated with moisture. To assess the pathogenicity of S. sclerotiorum isolates, the following scale was used (Table 2), which reflects the surface area of the affected leaf blade tissue (Table 2). Statistical analyses of research results were performed in Microsoft Excel.
Impact score | Lesion surface area, CM2 | Degree of pathogenicity |
---|---|---|
0 | 0 | Absent |
1 | <1 | Low |
2 | 1.1-2.0 | Medium |
3 | 2.1-2.9 | High |
4 | >3.0 | Very high |
Table 2. Scale used for assessing pathogenicity of S. sclerotiorum isolates.
Results and Discussion
Analysis of S. sclerotiorum isolates obtained from different host plants (Figure 2) revealed differences in their ability to produce sclerotia, with the number of sclerotia ranging from 8 to 36 pieces (pcs) per Petri dish. Based on the ability to produce sclerotia, S. sclerotiorum isolates were divided into two groups with moderate, from 8 to 21 pcs and intensive, from 23 to 36 pieces, productivity. Gm2, Han40, D26, Han41, Han39, Han 19 isolates were part of the latter group, while the remaining 36 isolates (Han14, Gm3, Han 18, Bn29, Bn31, Han16, Gm4, Han13, Ps36, Gm7, D23, D25, D27, Gm1, Han12, Han20, Bn30, Bn32, Ps33, Gm5, Han9, Han11 , Han15, Han17, D21, Bn28, Ps37, Han38, Han8, Ps34, Ps35, Han42, Gm6, Han10, D24, D22) produced from 8 to 21 sclerotia in the area of the nutrient medium of Petri dish. Most isolate that intensively produced sclerotia belonged to the population of S. sclerotiorum isolated from sunflower plants in the Kyiv region, Yahotyn district, which indicates a high potential for accumulation of infectious material.
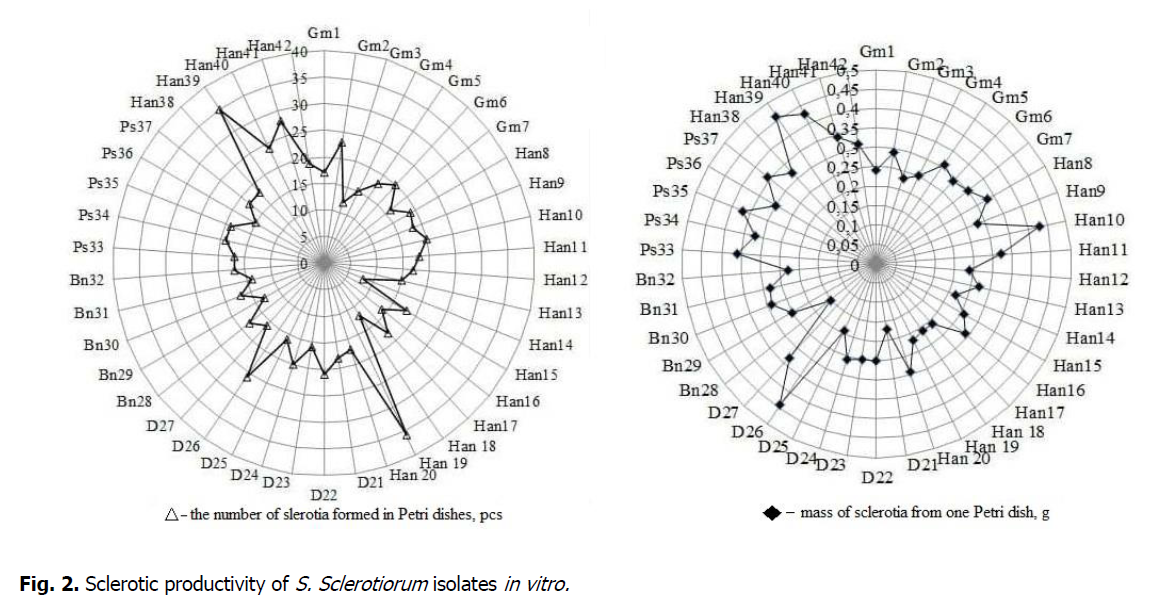
Figure 2: Sclerotic productivity of S. Sclerotiorum isolates in vitro.
As for the mass of sclerotia produced by isolates, the figure ranged from 0.15 g to 0.46 g. Bn28 produced the smallest mass of sclerotia (0.15 g), D21 (0.17 g), and D25 (0.19 g) isolates, while the largest - by Han10 and Han 40 (0.43 g), D26 (0.44 g), and Han 39 (0.46 g) isolates. For all other studied isolates of S. sclerotiorum, the mass of sclerotia was in the range of 0.21-0.37 g per Petri dish.
Significant differences were found in the different combinations of S. sclerotiorum isolates (Figure 3). Isolates obtained from soybean plants (population A, Kyiv region) had the lowest level of mycelial compatibility - 14%. Slightly higher compatibility, 29%, was observed in fungal isolates obtained from sunflower plants (population B, Zhytomyr region). The number of isolates that exhibited mycelial compatibility in populations E (winter rape, Kyiv region), G (sunflower, Kyiv region, Yahotyn district), D (dahlia, Kyiv), and F (peas, Ivano-Frankivsk region) was 35%, 38%, 48%, and 49%, respectively. In population C (sunflower, Kyiv region, Bila Tserkva district), the level of mycelial compatibility was the highest, and it was observed in 67% of isolate pairs.
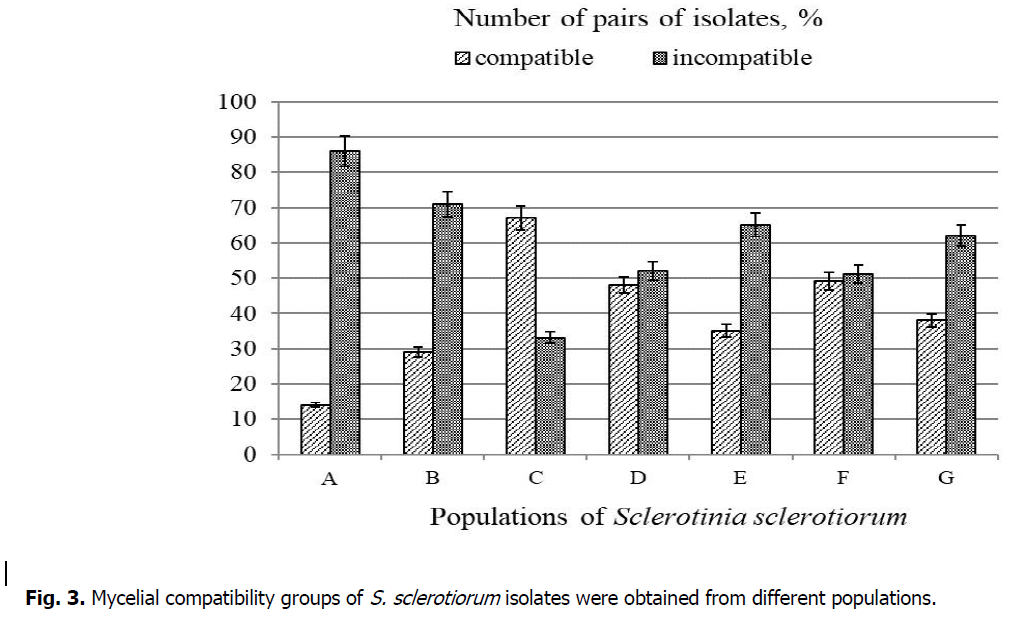
Figure 3: Mycelial compatibility groups of S. sclerotiorum isolates were obtained from different populations.
All 42 studied isolates of S. sclerotiorum obtained from different host plants caused damage to the leaves of winter rape and led to pathological processes (Figure 4). The lowest degree of pathogenicity was shown by Han42, Ps37, D21, and Han38 isolates, with lesion surface area on the leaf blade ranging from 0.7 to 1.0 cm2. Han8, Han12, Han17, Han16, Gm6, Bn28, and Ps33 isolates exhibited moderate pathogenicity. The following isolates of S. sclerotiorum showed strong pathogenicity: Han18, D24, Gm2, Ha13, D22, D27, Han14, Ps35, Ps35, Han9, Han11, D23, D26, Han39, Gm5, Han20, and Bn32. Han40, Han10, Gm3, Gm7, Han19, Han41, D25, Bn31, Gm1, Ps34, Gm4, Bn29, and Bn30 isolates had a very high degree of pathogenicity.
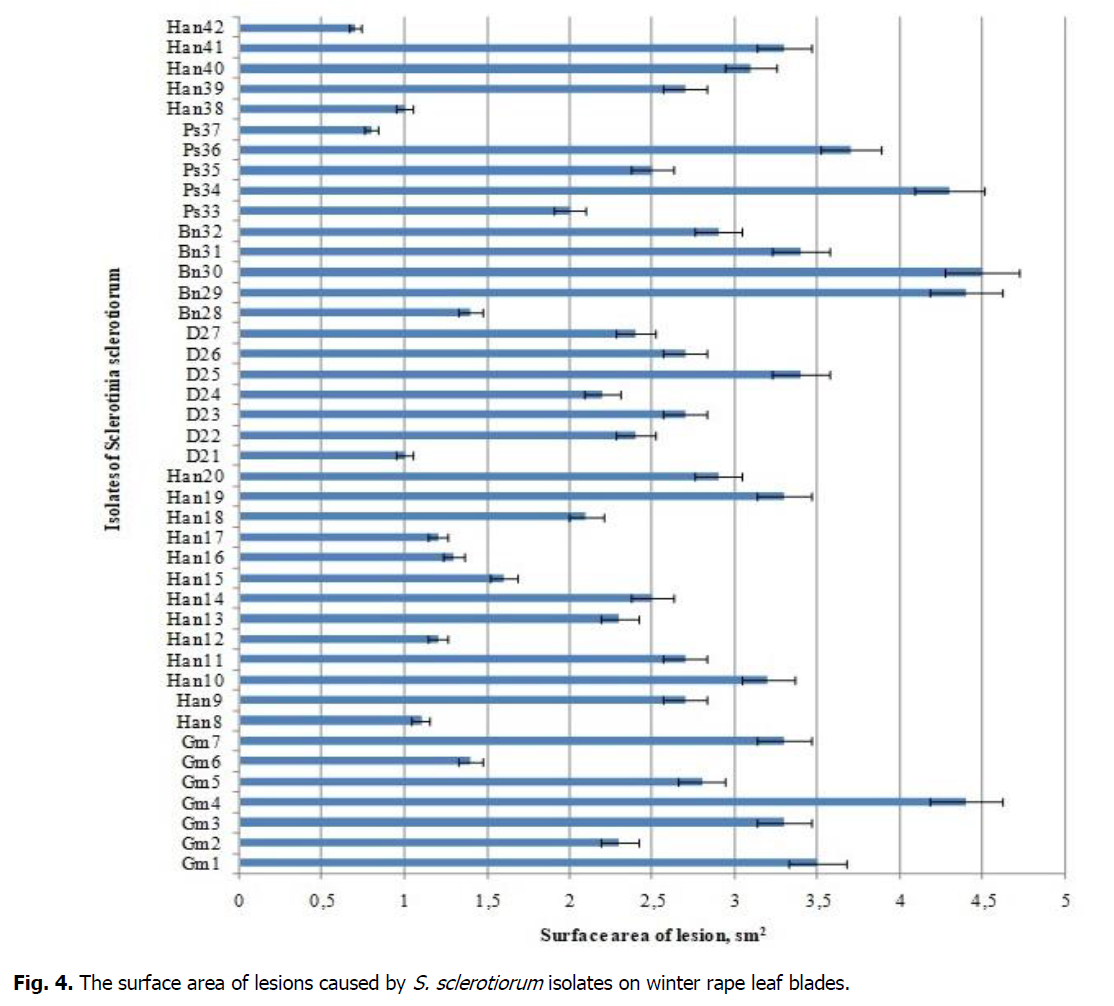
Figure 4: The surface area of lesions caused by S. sclerotiorum isolates on winter rape leaf blades.
The relationship between the number of sclerotia, their mass, and the surface area of lesions on leaf blades was expressed using a linear equation and the coefficient of determination (R2). Linear correlation was calculated to be statistically significant (10% significance level). It was found that the surface area of leaf blade lesions was positively correlated with the number of sclerotia in sunflower isolates in populations B (Y=0.8+0.07X, R2=0.42) and E (Y=-1.0+0.13X, R2=0.53). The coefficient of determination of the number of sclerotia was 42 to 53%, respectively, with the surface area of lesions on the leaf blades. In S. sclerotiorum isolates from population A, the surface area of leaf blade lesions decreased with an increase in the number of sclerotia produced (Y=6.2- 0.18X, R2=0.46). The surface area of leaf blade lesions decreased with an increase in sclerotia mass in population F (Y=14.7-35.2X, R2=0.59) and increased with an increase in sclerotia mass in population E (Y=-1.5+20.2X, R2=0.78). The mass of sclerotia ranged from 59% to 78% of the determination with the surface area of leaf blade lesions.
Comparison of pathogenicity levels between populations of S. sclerotiorum obtained from different geographical conditions and host plants showed a difference in the aggressiveness potential of studied isolates (Figure 5). In populations of A, B, C, and E, no isolates with weak pathogenicity were found, while their number in the populations D, F, and G was 14.3%, 20, and 40%, respectively. Isolates with moderate pathogenicity constituted half of the population C. Most isolates in populations B (57.1%) and D (71.4%) had strong pathogenicity. In turn, isolates with very strong pathogenicity prevailed in populations F and G (40%), A (57.1%) and E (60%).
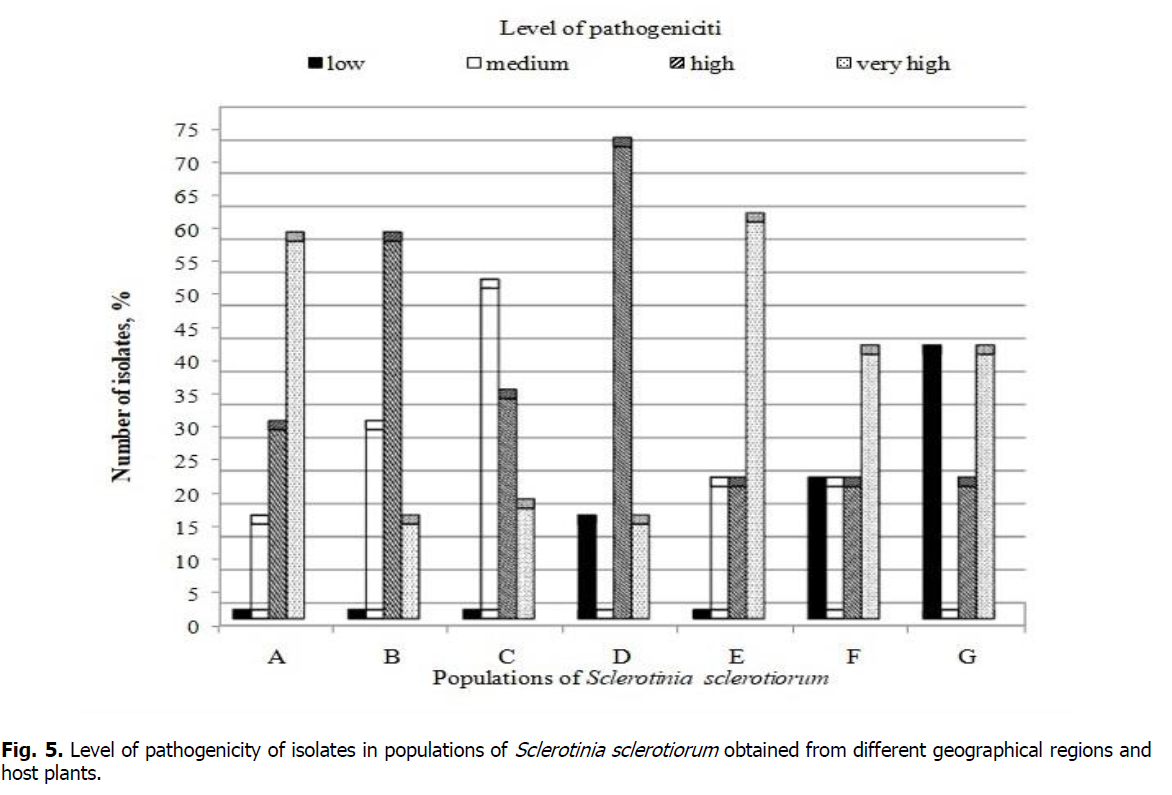
Figure 5: Level of pathogenicity of isolates in populations of Sclerotinia sclerotiorum obtained from different geographical regions and host plants.
All 42 investigated isolates of S. sclerotiorum obtained from soybean, sunflower, dahlia, rape, and pea crops, including plants from geographically remote regions, affected the test object, leaf of winter rape lack of pathogen specialization. The lack of convincing evidence for the existence of S. sclerotiorum specialization for host plants was also highlighted by Kull L. and co-authors (Kull et al., 2004). Differences in mycelial compatibility groups and changes in pathogenicity of S. sclerotiorum isolates may be responsible for different results of the evaluation of varieties' resistance to white mold in vitro and the field (Kim et al., 1999). In our studies, the level of antagonistic relationships in mycelial compatibility tests ranged from 33% to 86%, which indicates a high level of pathogen diversity. The isolates with very strong pathogenicity, which dominated in populations of S. sclerotiorum from pea plants (Ivano- Frankivsk region, Horodenka district), sunflower (Kyiv region, Yahotyn district), soybean, and winter rape (Kyiv region, Vasylkiv district), should be considered for the creation of artificial infectious backgrounds. It is advisable to use several isolates and isolates of S. sclerotiorum from the population obtained from sunflower plants with a high potential for sclerotia production (Kyiv region, Yahotyn district) can also be used.
Conclusion
Studied populations of fungus S. sclerotiorum were highly variable in their ability to produce sclerotia. The most significant numbers of sclerotia were produced by Gm2, Han40, D26, Han41, Han39, and Han19 isolates - from 23 to 36 pieces. Han10 and Han 40 (0.43 g), D26 (0.44 g), and Han 39 (0.46 g) isolate had the highest productivity in terms of accumulation of sclerotia mass. The study results revealed that the fraction of compatible pairs of isolates in different populations ranged from 14 to 67%. Comparison of pathogenicity levels between isolates in populations of S. sclerotiorum obtained from different geographical conditions and host plants showed differences in the aggressiveness potential. In populations of A, B, C, and E, no isolates with weak pathogenicity were found, while their number in the populations D, F, and G was 14.3%, 20%, and 40%, respectively. Isolates with moderate pathogenicity constituted half of population C. Most isolates in populations B (57.1%) and D (71.4%) had strong pathogenicity; whist isolates with very strong pathogenicity prevailed in populations F and G (40%), A (57.1%), and E (60%). These results emphasize the need to monitor pathogenicity in populations of a causative agent of white mold in areas of cultivation of susceptible crops, which will contribute to the development of effective disease control strategies.
References
Aldrich-Wolfe, L., Travers, S., & Nelson Jr, B.D. (2015). Genetic variation of Sclerotinia sclerotiorum from multiple crops in the North Central United States. PLoS One, 10:e0139188.
Attanayake, R.N., Tennekoon, V., Johnson, D.A., Porter, L.D., del Río-Mendoza, L., Jiang, D., Chen, W. (2014). Inferring outcrossing in the homothallic fungus Sclerotinia sclerotiorum using linkage disequilibrium decay. Heredity, 113:353-363.
Barro, J.P., Meyer, M.C., Godoy, C.V., Dias, A.R., Utiamada, C.M., Jaccoud Filho, D.D.S., Del Ponte, E.M. (2019). Performance and profitability of fungicides for managing soybean white mold: a 10-year summary of cooperative trials. Plant Disease, 103:2212-2220. Boland, G.J., Hall, R. (1994). Index of Plant Hosts of Sclerotinia sclerotiorum. Canadian Journal of Plant Pathology, 16:93-108. Boroday, V.V., Kirik, N.N., Koltunov, V.A. (2001). Pathological changes in roots of carrot causal by fungi during the storage. Mikologiia I Fitopatologiia, 35:48-51.
Carbone, I., Anderson, J. B., Kohn, L.M. (1999). Patterns of descent in clonal lineages and their multilocus fingerprints are resolved with combined gene genealogies. Evolution, 53:11-21.
Derbyshire, M.C., Denton-Giles, M. (2016). The control of sclerotinia stem rot on oilseed rape (Brassica napus): current practices and future opportunities. Plant Pathology, 65:859-877.
Foley, M.E., Dogramaci, M., West, M., Underwood, W.R. (2016). Environmental factors for germination of Sclerotinia sclerotiorum sclerotia. Journal of Plant Pathology Microbiology, 7:379-383.
Gulya, T., Harveson, R., Mathew, F., Block, C., Thompson, S., Kandel, H., Markell, S. (2019). Comprehensive disease survey of US sunflower: Disease trends, research priorities and unanticipated impacts. Plant Disease, 103:601-618.
Hemmati, R., Javan-Nikkhah, M., Linde, C.C. (2009). Population genetic structure of Sclerotinia sclerotiorum on canola in Iran. European Journal of Plant Pathology, 125:617-628.
Kim, H. S., Sneller, C. H., Diers, B. W. (1999). Evaluation of soybean cultivars for resistance to Sclerotinia stem rot in field environments. Crop Science, 39:64-68.
Kohn, L.M., Carbone, I., Anderson, J.B. (1990). Mycelial interactions inSclerotinia sclerotiorum. Experimental mycology, 14:255-267. Kull, L.S., Pedersen, W.L., Palmquist, D., Hartman, G.L. (2004). Mycelial compatibility grouping and aggressiveness of Sclerotinia sclerotiorum. Plant Disease, 88:325-332.
Lane, D., Denton-Giles, M., Derbyshire, M., Kamphuis, L. (2019). Abiotic conditions governing the myceliogenic germination of Sclerotinia sclerotiorum allowing the basal infection of Brassica napus. Australasian Plant Pathology, 48:1-7.
Lane, D.W., Kamphuis, L.G., Derbyshire, M.C., Denton-Giles, M. (2018). Heat-dried sclerotia of Sclerotinia sclerotiorum myceliogenically germinate in water and are able to infect Brassica napus. Crop and Pasture Science, 69:765-774.
Lehner, M.S., Lima, R.C, Carnciro, J.E.S., Paula Junior, T.J., Vieira, R.F., Mizubuti , E.S.G. (2016). Similar Aggressiveness of Phenotypically and Genotypically Distinct Isolates of Sclerotinia sclerotiorum. Plant Disease, 100:360–366.
Liu, J.X., Qinglin, M., Yunhua, Z., Hongtao, X., Yichu, L., Fengmei, S., Ligong, M., Chunlai, L., Yu, L., Baohua, S., Zhiyong, L. (2018). Mycelial compatibility group and genetic variation of sunflower Sclerotinia sclerotiorum in Northeast China. Physiological and Molecular Plant Pathology, 102:185-192.
Mamadou, F., Boyse, J., Wang, D., Willbur, J., Smith, D., Chilvers, M. (2017). Case Study of an Epidemiological Approach Dissecting Historical Soybean Sclerotinia Stem Rot Observations and Identifying Environmental Predictors of Epidemics and Yield Loss. Phytopathology, 108:469-478.
Morrall, R., Duczek, L., Sheard, J. (2011). Variations and correlations within and between morphology, pathogenicity, and pectolytic enzyme activity in Sclerotinia from Saskatchewan. Canadian Journal of Botany, 50:767-786.
Pemberton, I.J., McLaughlin, M.R., Smith, G.R. (1991). Inheritance of resistance to virus-induced lethal wilt in arrowleaf clover. Phytopathology, 81:1001-1005.
Pikovskyi, M., Kolesnichenko, O., Melnyk, V., Serediuk, O. (2019). Parasitism of micromycete Sclerotinia sclerotiorum (Lib.) de Bary on the dahlia plants (Dahlia cav.) under different weather conditions. Bìoresursi ì prirodokoristuvannâ, 11:16-24.
Rahman, M.M.E., Suzuki, K., Islam, M.M., Dey, T.K., Harada, N., Hossain, D.M. (2020). Molecular characterization, mycelial compatibility grouping, and aggressiveness of a newly emerging phytopathogen, Sclerotinia sclerotiorum, causing white mold disease in new host crops in Bangladesh. Journal of Plant Pathology, 102.
Rathi, A.S, Minakshi, J., Rakesh, P., Subaran, S., Pawan, K., Ram, A. (2018). Morphological and molecular diversity of Sclerotinia sclerotiorum infecting Indian mustard. Indian Phytopathology, 71:407-413.
Schafer, R.S, Kohn, L.M. (2006). An Optimized Method for Mycelial Compatibility Testing in Sclerotinia sclerotiorum. Mycologia, 98:593–597.
Sharma, P., Samkumar, A., Rao, M., Singh, V.V., Prasad, L., Mishra, D.C., Gupta, N.C. (2018). Genetic diversity studies based on morphological variability, pathogenicity and molecular phylogeny of the Sclerotinia sclerotiorum population from Indian mustard (Brassica juncea). Frontiers in Microbiology, 9:1169.
Tok, F.M., Dervis, S., Arslan, M. (2016). Analysis of genetic diversity of Sclerotinia sclerotiorum from eggplant by mycelial compatibility, random amplification of polymorphic DNA (RAPD) and simple sequence repeat (SSR) analyses. Biotechnology & Biotechnological Equipment, 30:921-928.
Willbur, J., Mamadou, F., Bloomingdale, C., Byrne, A., Chapman, S., Isard, S., Magarey, R., McCaghey, M., Mueller, B., Russo, J., Schlegel, J., Chilvers, M., Mueller, D., Kabbage, M., Smith, D. (2017). Weather-Based Models for Assessing the Risk of Sclerotinia sclerotiorum Apothecial Presence in Soybean (Glycine max) Fields. Plant Disease, 102:73–84.
Winton, L.M, Leiner, R.H, Krohn, A.L. (2006). Genetic Diversity of Sclerotinia species from Alaskan Vegetable Crops. Canadian Journal of Plant Pathology, 28:426-434.
Zanatta, T., Kulczynski, S., Guterres, C., Fontana, D., Meira, D., Ceolin, E., Balem, E., Trevisan, M., Paraginski, J., Buffon, P. (2019). Morphological and Patogenic Characterization of Sclerotinia sclerotiorum. Journal of Agricultural Science, 11:302-313.
Zhang, Z., Zhang, X., Liu, F., Mu, W. (2015). Insecticide susceptibility of the green plant bug, Apolygus lucorum Meyer-Dur (Homoptera: Miridae) and two predatory arthropods. Journal of Plant Protection Research, 55:354-361.
Author Info
M. Kyryk1, M. Pikovskyi1, O. Kolesnichenko1, V. Borodai1, O. Markovska2*, V. Dudchenko2 and M. Solomiyciuk32Kherson State Agrarian and Economic University, Stritenska St. 23, 73006, Kherson, Ukraine
3Ukrainian Scientific-Research Plant Quarantine Station, Institute of Plant Protection, NAAS 1, Naukova Street, v. Boyani, Novoselytsa district, Chernivtsi region, 60321, Ukraine
Citation: Kyryk, M., Pikovskyi, M., Kolesnichenko, O., Borodai, V., Markovska, O., Dudchenko, V., Solomiyciuk, M. (2021). Sclerotic productivity, mycelial compatibility and pathogenicity of the isolates of the fungus Sclerotinia sclerotiorum (Lib.) de Bary. Ukrainian Journal of Ecology, 11 (5), 21-28.
Received: 15-Jun-2021 Accepted: 25-Jun-2021 Published: 29-Jul-2021
Copyright: This is an open access article distributed under the terms of the Creative Commons Attribution License, which permits unrestricted use, distribution, and reproduction in any medium, provided the original work is properly cited.